Overview
Mechanics of nanoporous materials
This project centers on building a fundamental understanding of the deformation behavior of small-scale materials, with a focus on nanoporous (NP) materials, that contain fractionally many surfaces/interfaces. The macroscopic functionality of NP and other nano-scale materials hinges on the behavior of surfaces and interfaces. In my group, we develop transformational computational modeling and simulation methods to realize fundamentals of matter behavior that originate at small scales and at surfaces/interfaces but bridge to multiscale properties of advanced bulk materials.
Computational prediction of the structure of semicoherent interfaces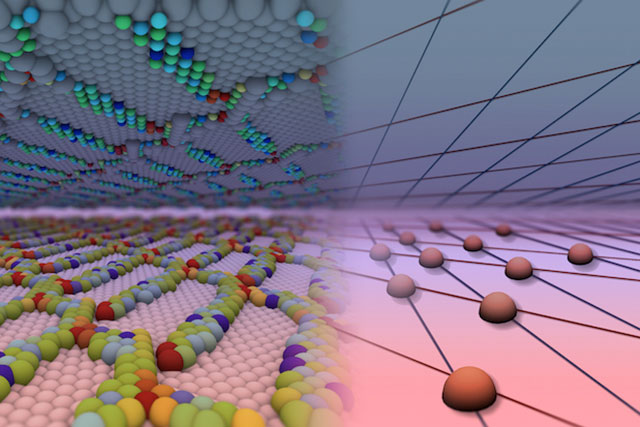
Solid-state interfaces are ubiquitous in materials science: from grain boundaries in polycrystalline metals to heterophase interfaces in multi-component, multi-functional composites . As awareness of their far-reaching influence on materials behavior grows, so does interest in predicting and controlling their structure and properties. In this project, we advance the dislocation-based model for interface structure by developing a method for determining the unique reference state in which interface Burgers vectors must be defined.
Deformation/Phase-Transformation Behaviors of Materials under Extreme Pressures
At extraordinary high pressures, like those naturally occurring at the earth’s core, materials can undergo extreme real-time structural changes. Recent experiments have discovered that the transformation time for many classes of phase transformations is less than 10-9 and in some cases 10-12 seconds. New phase nucleation/transformation processes however are poorly understood at rapid (nanoseconds or less) timescales due to temporal and spatial limitations of conventional pump-probe measurements. In-situ X-ray diffraction (XRD) crystallography is not sufficient to determine the exact phase transformation pathway from high-pressure high-temperature experiments since multiple transformation paths can potentially produce a similar final phase configuration. Here, with the aid of NEMD, we seek a fundamental understanding of the exact plastic deformation mechanisms and structural phase transformation pathways during the shock and ramp compressing of materials.
Automated Analysis of Big X-ray Diffraction Data
Getting a fundamental understanding of the mechanisms that govern the arrangement and motion of atoms is important for identifying new pathways for forming new matter with desired properties and behavior at extreme conditions. However, phase nucleation/transformation processes are poorly understood at rapid timescales due to the temporal and spatial limitations of conventional pump-probe measurements. Emerging X-ray scattering techniques provide the capability to simultaneously probing the correlations between structure and material properties with sensitivity approaching the single molecule level and picosecond resolution. Temporal diffraction images are data-intensive and manually analyzing large experimental diffraction datasets with many uncertainties cannot solely be performed by human experts. At high pressures, when the lattice variations or occurrence of new phases is not known a priori, analyzing vast datasets of snapshots from billions of XRD measurements becomes even more inaccurate, or fail completely. Thus, it is crucial to automate the image processing workflow as much as possible to generate fundamental and accurate knowledge. During this project, we develop automated deep-learning techniques to mine information-rich XRD data and filter and detect lattice-level mechanisms responsible for phase transformation and plastic deformation under extreme conditions.