Microbial Engineering
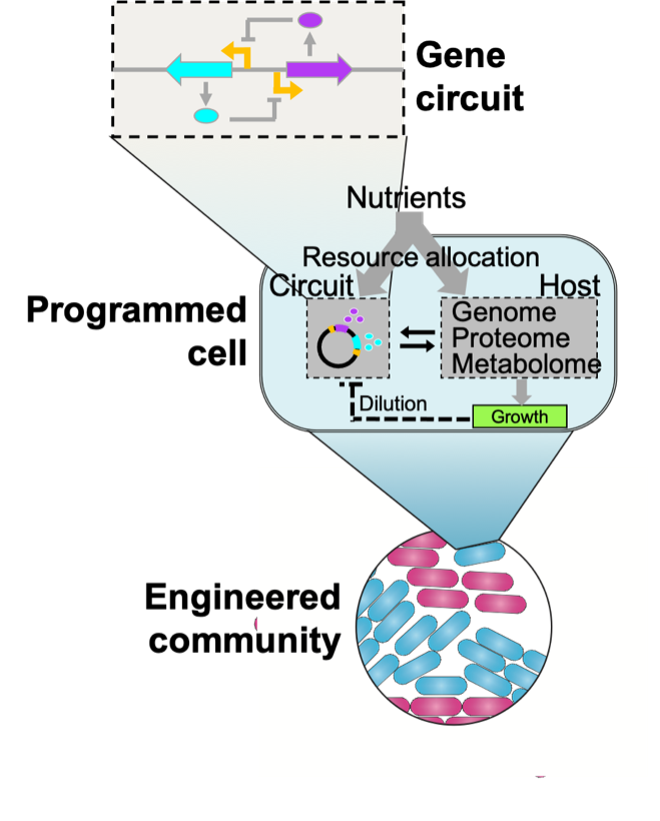
Microbial communities are found in virtually every unique environmental niche on earth. In these communities, microbes perform a host of functions that are vital for human and environmental health, ranging from microbes in our gut that help us digest foods and produce vitamins, all the way to microbes in the oceans that help degrade crude oil contamination. Microbes can also pose significant threats—pathogenic bacteria are responsible for a variety of infectious diseases. In general, understanding and leveraging microbial processes has massive therapeutic, industrial and biotechnological implications. Indeed, microbes and microbial communities can be engineered using gene circuits, or pieces of DNA (or members of a community) arranged in a purposeful way to achieve a desired function. However, a key challenge in microbial engineering is achieving robust and reproducible function given the inherent complexity and variability of biological systems. Microbial engineering therefore depends as much on understanding the underlying biomolecular components as it does on adequate mathematical models of the desired behaviors; this intersection between microbiology, mathematical modeling, and biomolecular engineering is the cutting edge of systems and synthetic biology within chemical engineering. These approaches are of particular interest in the study of antibiotic resistance; ongoing work in the department focuses on leveraging biological insights and engineering approaches to design microbial communities and therapeutic strategies capable of slowing the spread of resistance genes.
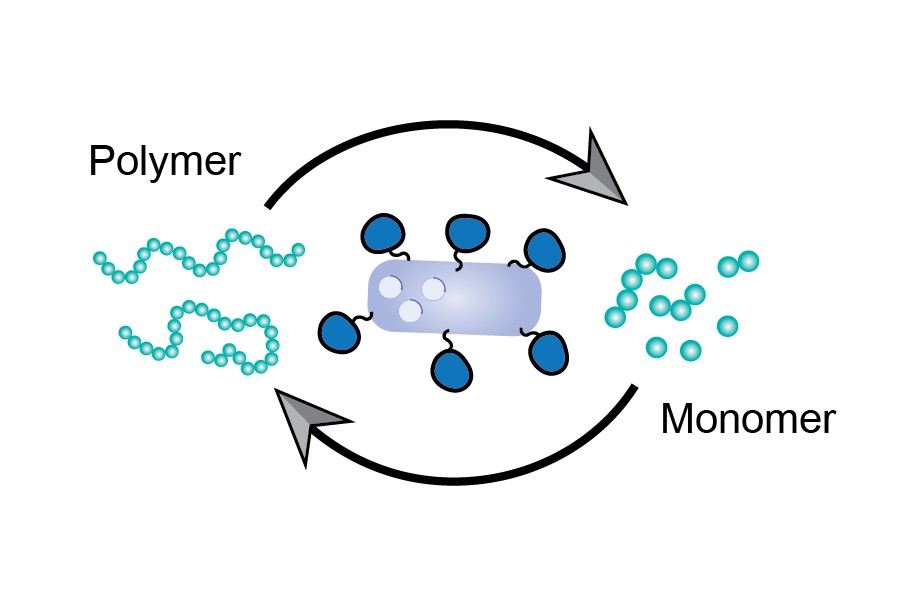
Microbial engineering involves the manipulation of microbes to develop new uses for them. Chemical engineering plays a pivotal role in the delivery of biological discovery and innovation for the benefit of society. In particular, microorganisms have become an increasingly important platform for the production of chemicals, drugs, and biofuels from renewable resources. Historically, plastics (polymers) were developed to minimize cost, maximize durability, and optimize performance rather than recyclability and reuse potential. Meanwhile, conventional polymerizations often rely on organic solvents and heavy metal catalysts that are contrary to sustainability goals. Our failure to address these issues in the inherent design of plastics combined with our global dependence on them has caused severe pollution and accelerated the depletion of natural resources. For the environmentally-friendly synthesis of polymers with defined sequences, we can take inspiration from microbes, which have synthesized sequence-controlled polymers in the form of proteins, polysaccharides, and nucleic acids for millions of years. The combination of microbial and chemical engineering offers a promising approach to improve the polymer industry and enable the development of greener plastics. This way, we can work towards a more sustainable and circular polymer ecosystem.
Active Faculty / Research Areas
Lopatkin: Systems biology, synthetic biology, computational biology, horizontal gene transfer, bacterial population dynamics, microbial metabolism, antibiotic resistance
Fan: Polymer chemistry, plastic upcycling, bio-inspired catalysis, synthetic biology, metabolic engineering, bioelectrochemistry and water-remediation.