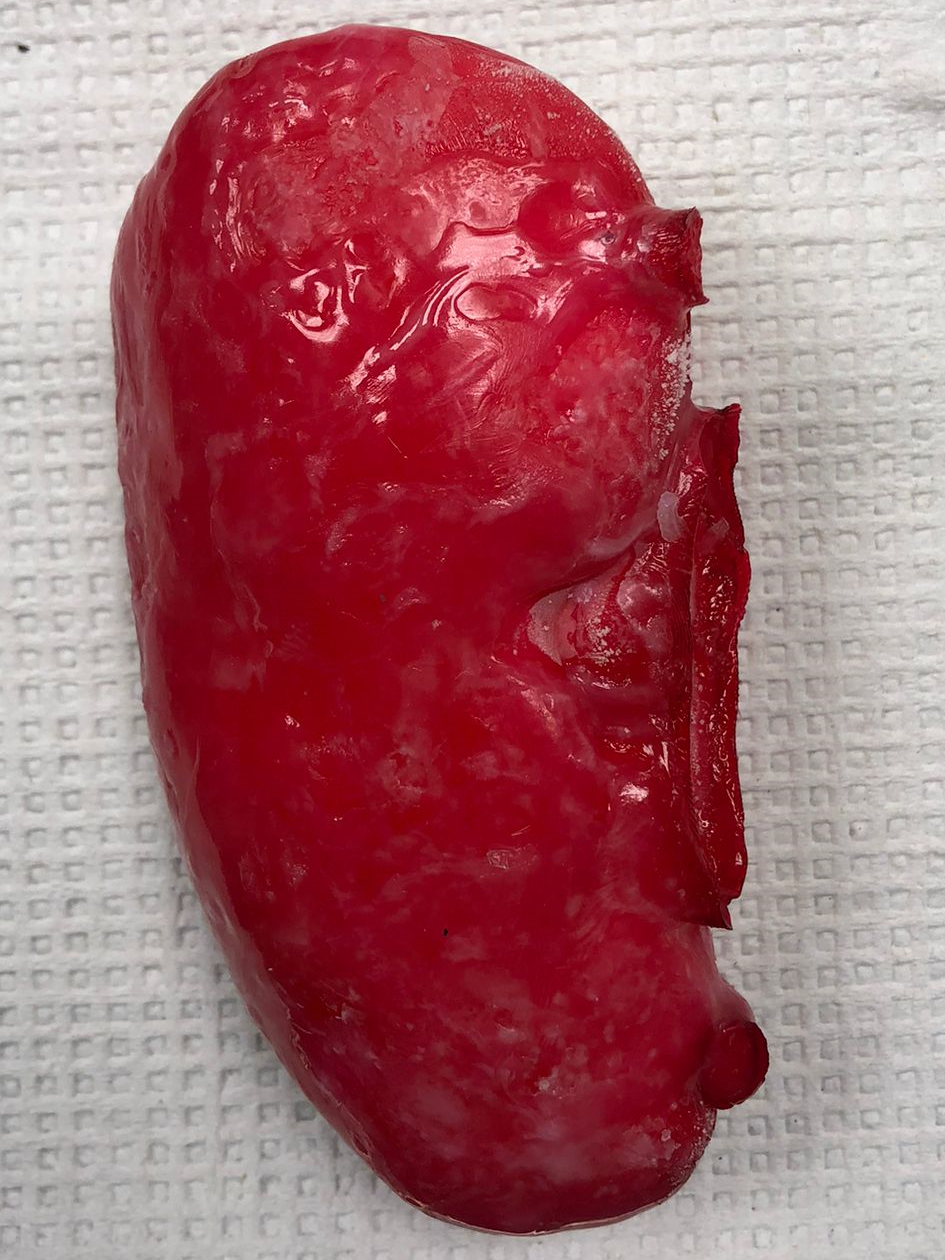
Introduction
URMC Kidney Model Optimization Project
Team Members:
Bimpe Isafiade, Harshita Mahaseth, Natalie Ramesh, Baris Eser Ugur
Project Sponsor:
Dr. Ahmed Ghazi
URMC Simulation Innovation Lab
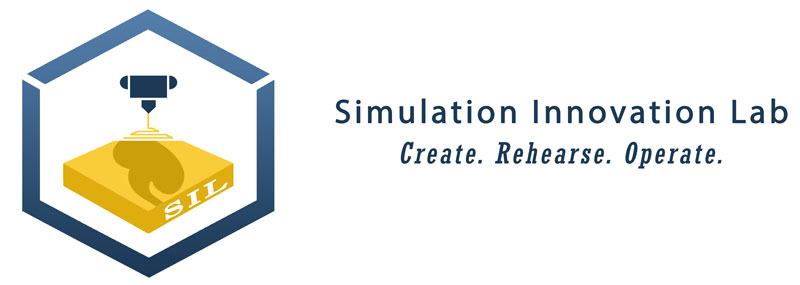

Campus Mentor:
Prof. Melodie Lawton
The main goal of this project is to optimize a procedure to synthesize PVA based polymers that mimic kidney tissue for residents to practice Percutaneous Nephrolihotomy (PCNL) surgery. The project is a unique combination of materials discovery & optimization, physical chemistry, process design and fundamentals of chemical engineering.
Project Background
The final polymer material needs to satisfy five various mechanical, chemical and optical requirements:
• The sample needs to have a 0-30% Strain Young’s Modulus value similar to that of the kidney, 0.05 MPa.
• The polymer material needs to have high IR Transparency at 850 nm while being opaque under visible light.
• The material needs to demonstrate no shrinkage due to phase separation within 48 hours.
• Upon insertion of a needle through the polymer, there should be no visible needle tracks at the surface and in the bulk of the material.
• The polymer should have a wide flexibility in its mechanical properties to model several tissues with varying Young’s Modulus values. The desired tissues are the skin, muscle, fascia, and the filler fat tissue in addition to the kidney.
Technical Approach
To successfully fulfill the mechanical, optical and chemical requirements of the materials, two main approaches were taken:
1. Re-molding of the polymer-rich phase after separation
Re-molding procedure was conducted through isolation of the polymer-rich phase after phase separation was completed. The isolated phase was melted and re-molded with a 3D printed kidney mold, quenched for an hour, and was left to crosslink for 12 hours. The dye was applied afterwards for reduced visible light transmission and to mimic a realistic surface and texture.

2. Synthesis through Original Procedure, Storage at -80 °C
With this procedure, the material was prepared through the original procedure involving 1 hour of quenching followed by 12 hours of crosslinking and dye addition. However, the crosslinked polymer was stored at -80 °C to slow down the kinetics of phase separation.
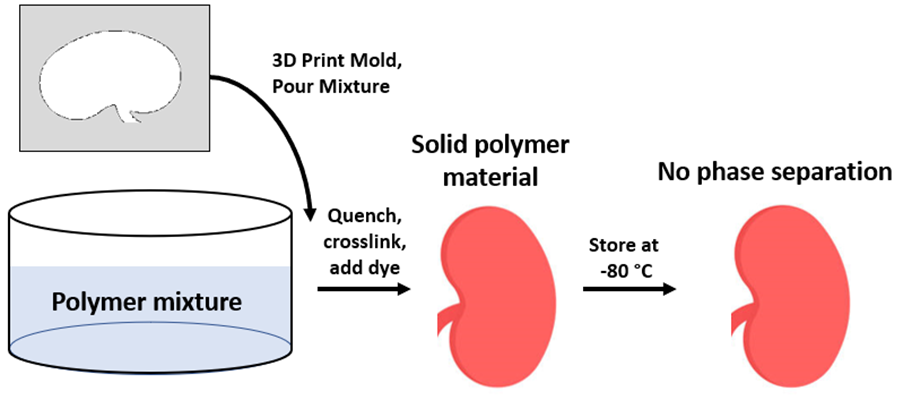
Two additional procedures were followed to eliminate the needle tracks, reduce visible light transmission and create a realistic texture:
3. Application of Water to Polymer Surface
Several droplets of distilled water were added to the polymer surface, and the visibility of tracks upon needle injection was evaluated for various surfaces.
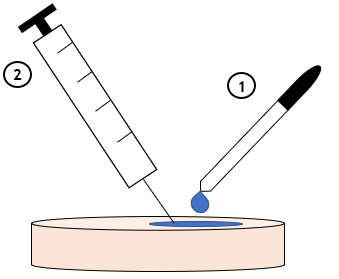
4. Addition of Water-Based Dye on Polymer Surface
Several drops of a cherry red water-based polymer were added to the polymer surface and mechanically dispersed. The dye was allowed to diffuse through the bulk over the span of several hours.
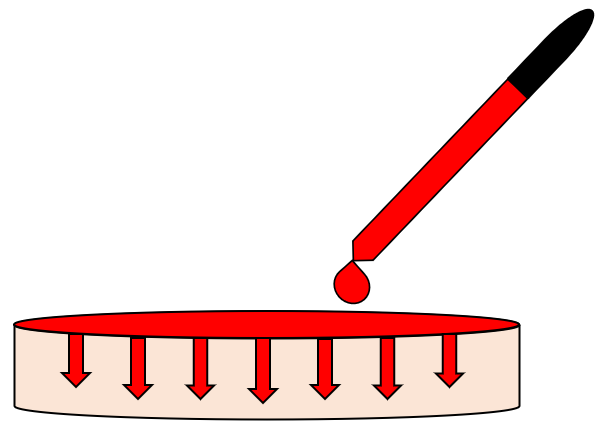
Results
Re-molding Procedure Results
The re-molding procedure was completed for various PVA compositions to investigate their optical, mechanical and chemical properties.
IR Transmission at 850 nm
• The sample demonstrated very high IR transmission at 850 nm as desired.
• Despite the measurement thickness of 5 cm, the metal surfaces were clearly visible, which is highly advantageous for surgical practice where a tumor needs to be located under IR light (simulating X-rays).
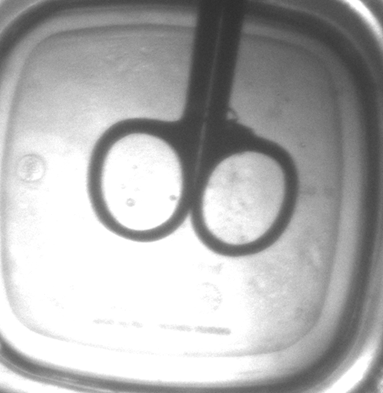
Figure 1. IR transmission of a 5 cm thick polymer sample prepared through re-molding.
Shrinkage Results after 48 Hours
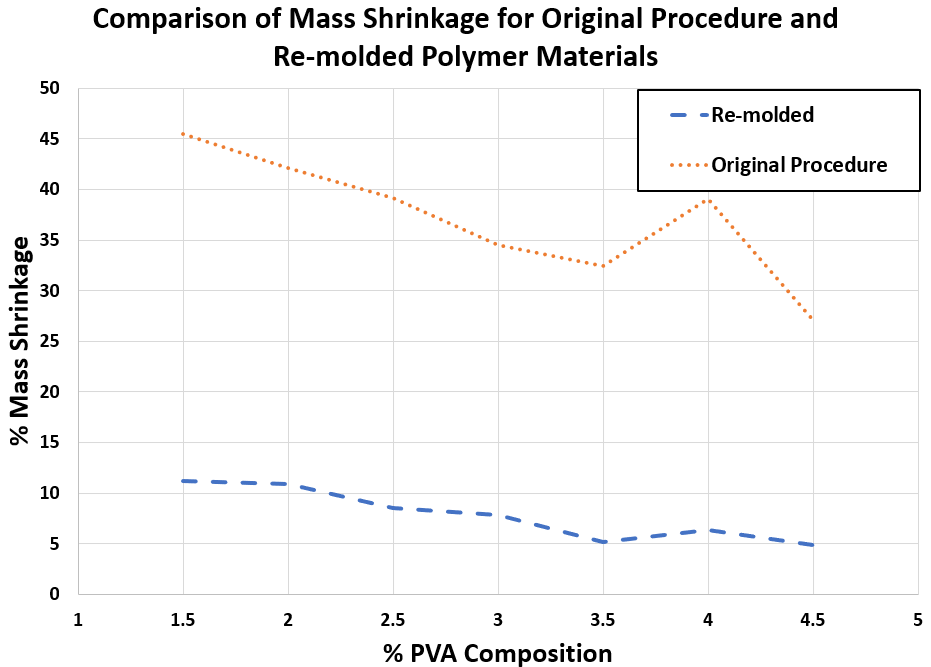
Figure 2. Comparison of % mass shrinkage for polymer samples synthesized through re-molding and the original procedure
• As described in Table 1, very low mass shrinkage was observed, which indicates that the re-molding procedure effectively prevents phase separation.
• Figure 2 highlights the decrease in mass shrinkage with the re-molding procedure compared to the original procedure.
• There was no observable volume shrinkage, which is the main priority of the chemical properties as the model organs should not change dimensions over time.
Table 1. Percent mass shrinkage of polymer samples with varying PVA composition, synthesized through re-molding.
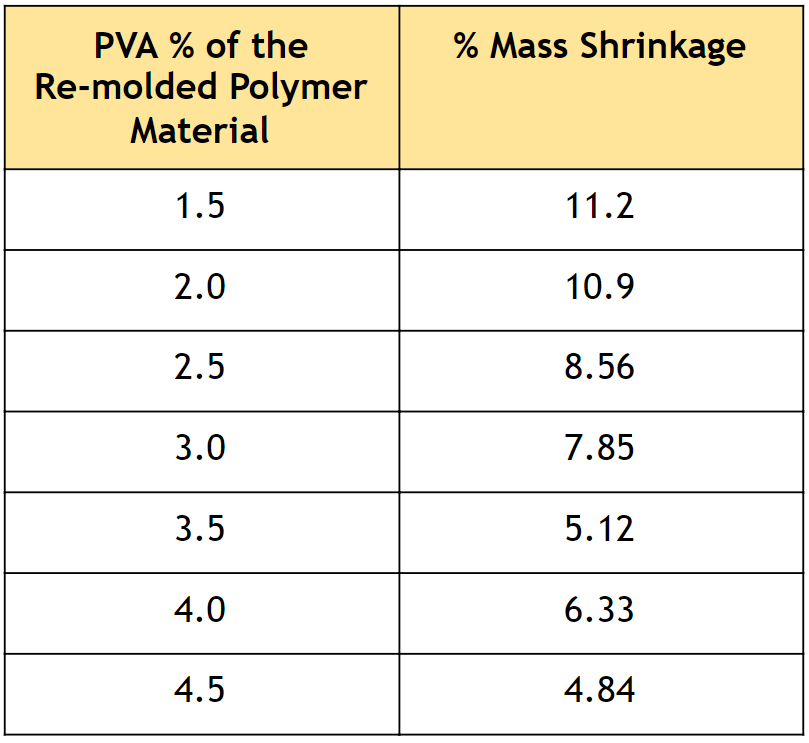
Young’s Modulus Results
• As visualized in Figure 3, the Young’s Modulus of the samples demonstrated a wide variety which is advantageous as various tissue layers can be modeled more accurately.
• By controlling the PVA composition of the polymer, the desired kidney properties can be achieved.
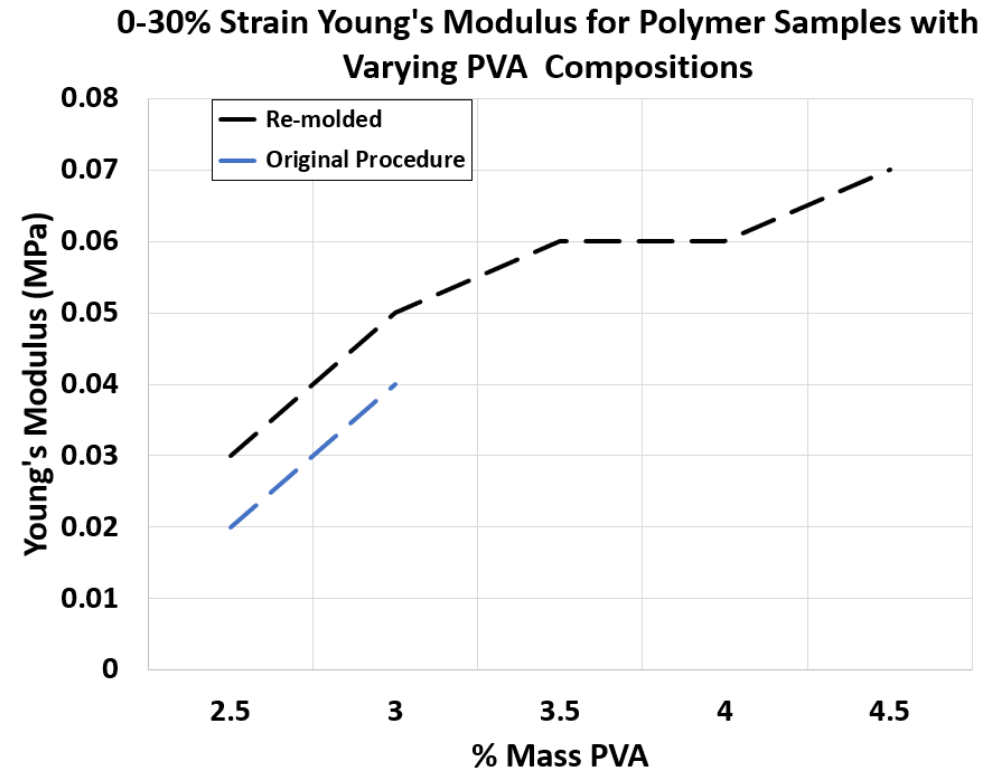
Figure 3. 0-30% Strain Young’s Modulus for Polymer Samples with Varying PVA Compositions synthesized through re-molding and original procedure
-80 °C Storage Procedure Results
IR Transmission at 850 nm
• Similar to the re-molding results, the -80 C results demonstrated very high IR transmission at 850 nm as desired.
• Again, despite the measurement thickness of 5 cm, the metal surfaces were clearly visible
• Comparison of IR properties of the two main approaches yield very similar results, indicating that storage at -80 °C does not affect the optical properties.
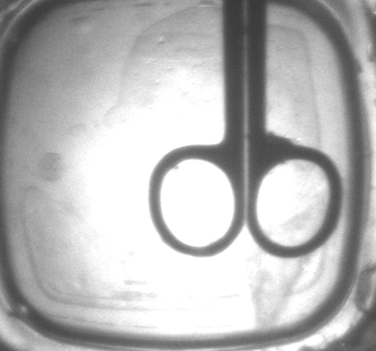
Figure 4. IR transmission of a 5 cm thick polymer sample prepared through -80°C storage procedure.
Shrinkage Results after 48 Hours
• There was no measurable mass shrinkage of the samples after 48 hours followed by full thawing of the sample.
• Similarly, no volume shrinkage was observed, indicating that the chemical specifications provided by the sponsor are fully satisfied.
• The phase separation was fully stopped with reduced kinetics at extremely low temperatures, which is further enhanced by the freezing of the solvents.
Young’s Modulus Results
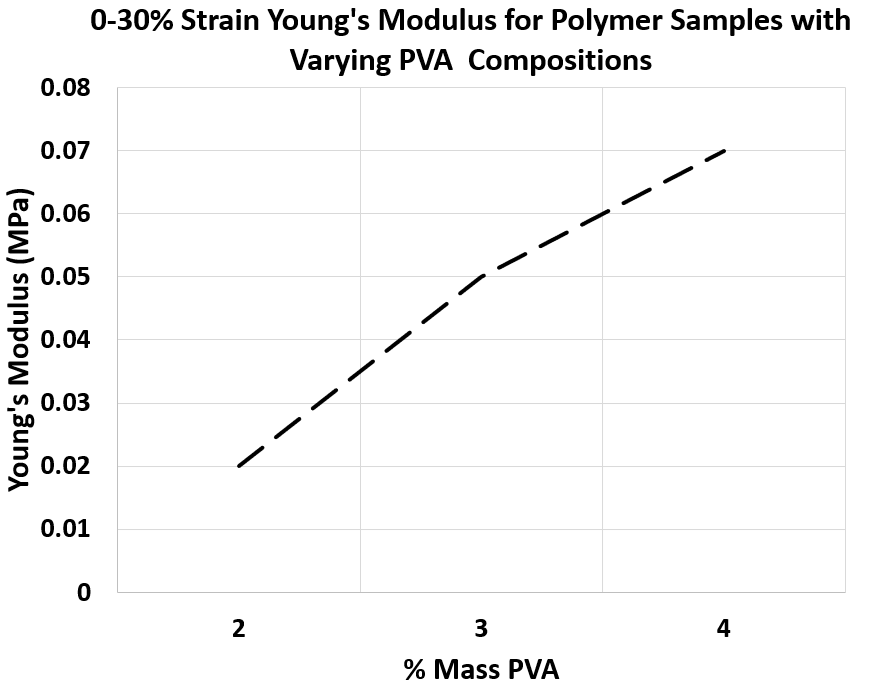
Figure 5. 0-30% Strain Young’s Modulus for Polymer Samples with Varying PVA Compositions synthesized with -80°C storage procedure
• Similar to the first approach, the Young’s Modulus of the samples has a wide variety as desired for modelling various tissue layers.
• As shown in Figure 5, the Young’s Modulus can be precisely controlled with varying PVA composition, which gives the ability to engineer different tissues accurately.
Needle Track Elimination Results
• Upon the addition of water on the polymer surface followed by needle insertion, no visible needle tracks at the surface or in the polymer bulk was observed.
• However, addition of water after needle insertion did not prevent the needle tracks, indicating the importance of order of the procedure.
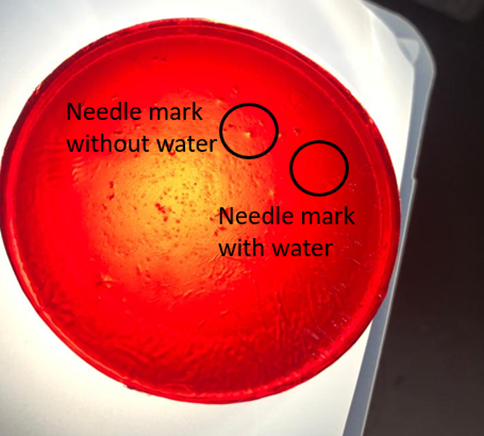
Figure 6. Comparison of needle tracks with and without water application for a polymer sample
Water-Based Dye Addition Results
• Post-synthesis water-based dye addition revealed very promising results as shown in Figure 7.
• Over time, a very uniform diffusion of the dye was achieved, which provided optical properties that did not vary with position.
• As the sample is shown under IR light at 850 nm, minimal negative impact on IR properties was observed, with the metal objects clearly visible through the material.
• With the dye addition, lowered visible light transmission was observed as desired.
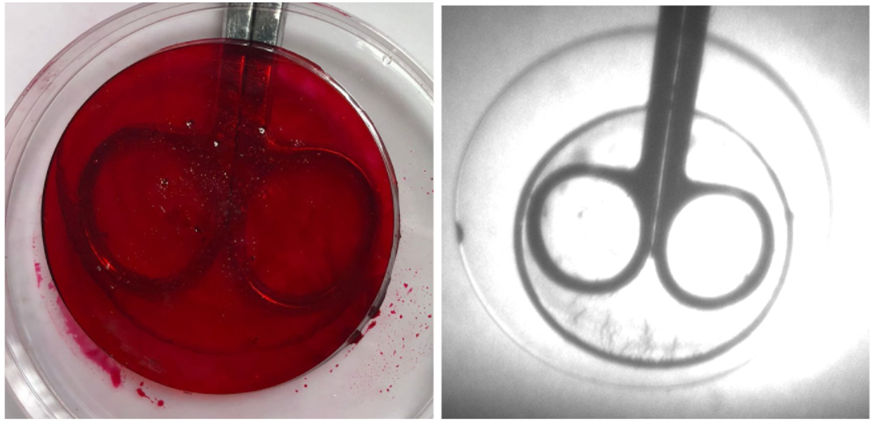
Figure 7. Polymer sample with water-based cherry red dye added (left) and polymer sample with water-based cherry red dye added under 850 nm IR light (right).
Final Prototype
Upon discussions with the sponsor, a final prototype with various layers of tissue types including the skin, fascia, filler fat, muscle and the kidney tissue was prepared.
All successful methods were combined:
• Re-molding
• Storage at -80°C
• Water-based dye addition
• Multiple tissues synthesized & assembled
The final prototype was tested for its optical and mechanical properties along with the evaluation of shrinkage over 48 hours.
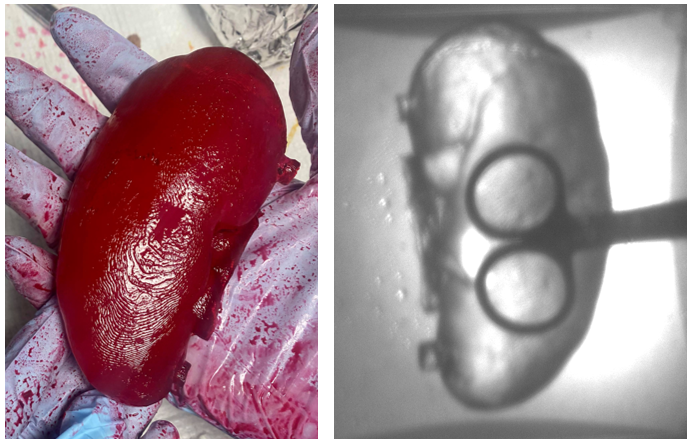
Figure 8. Picture of the final prototype under visible light (left) and IR light (right).
As shown in Figure 8, the final kidney prototype was extremely successful with a realistic texture. Furthermore, no shrinkage was observed after storage at -80 °C. As the IR transmission results indicate, the visibility through the material at 850 nm is still very high, which fulfills the optical specifications for the prototype.
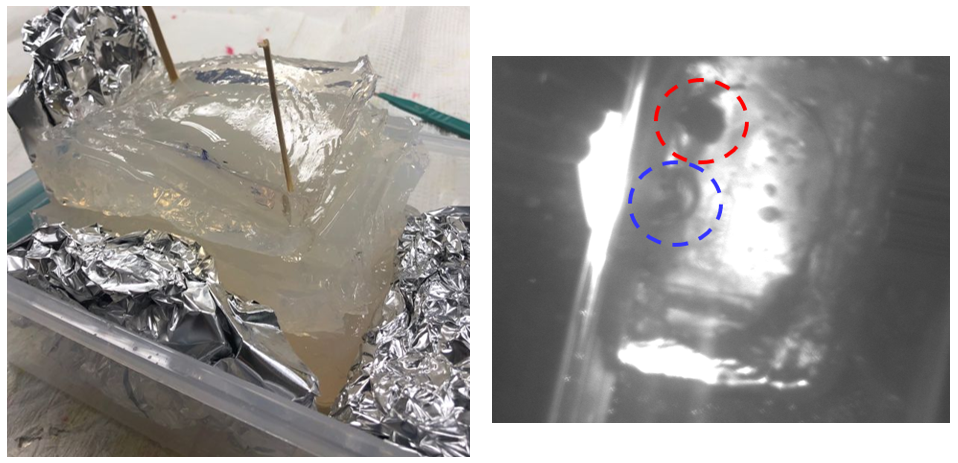
Figure 9. Picture of the multi-layer prototype (left) and the picture of the multi-layer prototype under IR light at 850 nm. The aluminum foil is circled in red, and the air gap is circled in blue.
The physical examination of the full prototype with the layers assembled indicated very positive results with Dr. Ghazi finding all tissues’ texture satisfactory. It was indicated that the kidney should be a little tougher, and two additional kidney models with higher PVA composition were synthesized to further improve its properties. As Figure 9 shows, the IR transmission of the prototype, which is approximately 15 cm, meets the specifications with the aluminum foil tumor model clearly visible at 850 nm (circled in red). Furthermore, it was observed that intentionally left air gaps are also visible under IR light (circled in blue), which indicates the potential for the simulation of the tumor tissue with air gaps if the tumor tissue needs to be penetrable.
Conclusions
• With a highly systematic approach, all five target properties were successfully achieved.
• The final prototype accurately simulated the tissues:
– Kidney, fascia, muscle, skin, filler fat
• High IR transmission for 15 cm thick prototype was achieved, indicating that the optical requirements are met.
• Realistic texture and low visible light transmission with water-based dye addition was observed, which further enhances the optical properties of the polymer material.
• With the water addition procedure, the needle tracks were successfully eliminated, allowing residents to practice repeatedly without being impacted by their previous practice runs.
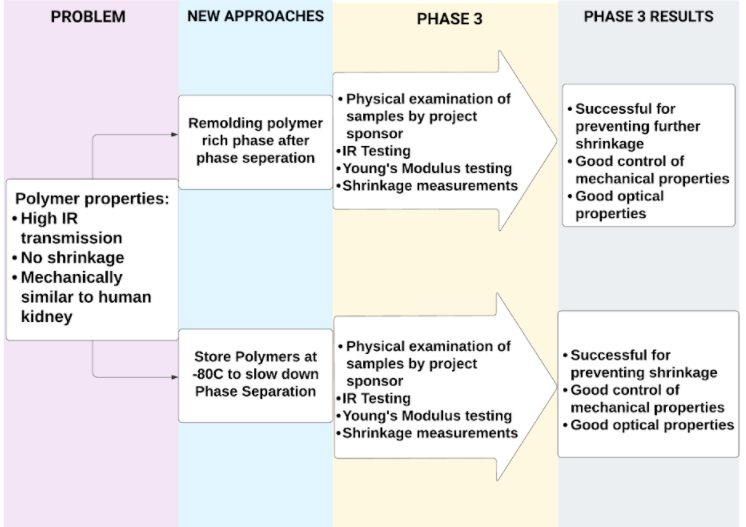
Future Work
Numerous areas can be further pursued to optimize the polymer material and its large-scale synthesis
- Reabsorption of water by shrunken samples should be evaluated as it would effectively reduce storage space and speed up the synthesis procedure.
- Optimization of either procedure for continuous synthesis:
– Design of protocol for the re-molding procedure to prevent any interruption in synthesis
– Procedure design for -80 °C storage approach to minimize storage space needs - Elimination of mechanical affects of needle insertion for long-term use of the models
- Economic optimization of the procedure to minimize DMSO use without impacting the mechanical, optical and chemical properties of the polymer materials
Acknowledgements
The team would like to thank Dr. Ahmed Ghazi, Prof. Melodie Lawton, Prof. Mark Juba, Prof. Doug Kelley, Rachel Monfredo, Jeff Lefler, Ryan Hayter, Clair Cunningham, Nathan Schuler, Tracy Bubel, Rachel Melnyk for their continuous support and help throughout the project.
References
• Young, T., & Chuang, W. (2002, October 22). Thermodynamic
analysis on the cononsolvency of poly (vinyl alcohol) in water–dmso mixtures through the ternary interaction parameter. Retrieved March 23, 2021, from
• Team Caladrius (2020). Kidney model polymer: Developing a stable model for surgical simulation. CHE 255. Retrieved February 16, 2021 from learn.rochester.edu.
• Team Phoenix (2020). Technology Transfer. CHE 255. Retrieved February 16, 2021 from learn.rochester.edu.