Biomedical Engineering
Senior Design Project 2022
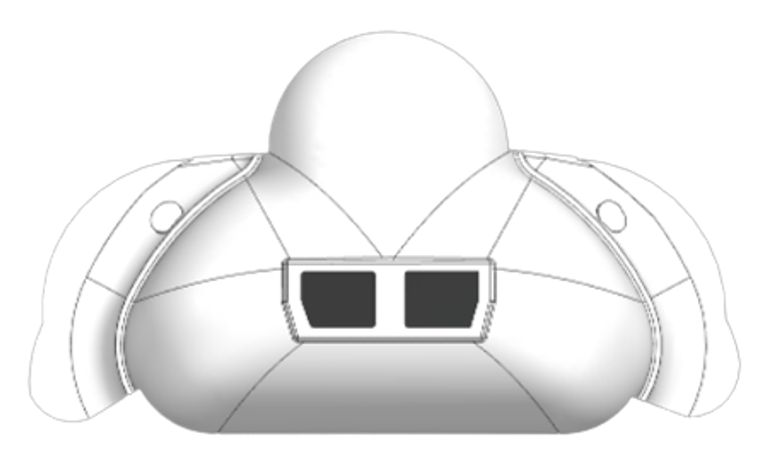
Problem Statement
Over 250,000 infants are born prematurely in the United States alone, and approximately 50,000 of them will develop Infant Respiratory Distress Syndrome (IRDS) (NORD, 2021). Continuous Positive Airway Pressure (CPAP) therapy is a therapeutic method used to treat IRDS where above-atmospheric pressure is administered to a patient through mask or prong interfaces. There are issues, however, with this system that impact the health of neonates. Neonates have delicate, underdeveloped skin, and thus are at an increased risk for developing pressure ulcers. CPAP masks can cause pressure injuries on the face and are prone to slipping which results in pressure leaks (Dylag, 2021). When a pressure leak forms, the neonate is no longer receiving the correct amount of oxygen, and since their lungs are underdeveloped this lack of oxygen and pressure will be dangerous. Currently, leaks can be detected through visual observation, drops in blood-oxygen saturation, or cues from the machine output; however, these three methods are risky for the infant and only able to detect significant leaks in the mask-patient interface (Dylag, 2021). Thus, there is a need to both detect the location of leaks that develop in the interface-infant seal and to reduce the pressure exerted on the neonate’s skin by the CPAP mask. Our improved mask was designed to address these issues with the addition of wings that increase the contact area at the neonate-mask interface to reduce the force exerted on the skin and utilizes gas pressure sensors to determine when and where an air leakage has developed.
Goals
Specification | Metric |
Neonate-Mask Interface Seal | Mask must sustain at least 85% of pressure input |
Reduction in Pressure from Mask to Infant | Mask must produce less pressure exerted on neonate’s face than current neonatal CPAP mask given equal loading conditions |
Detection of Possible Leaks in Neonate-Mask Interface | Mask must determine there is a break in the seal on either side where sensors are placed |
Adaption of Mask Dimensions for Multiple Sizes | Mask must be able to be adapted to fit a range of different sized babies |
Anthropometric Restrictions Regarding Eyes and Nose | Mask must not overlap eye sockets and mouth when properly placed |
Biocompatible | Mask must be stable under physiological conditions (98 °F and 100% humidity), not leach harmful chemicals to the infant, and comply with ISO 10993 Standard |
Compatible with Current Mask System in URMC NICU | Mask must interface with the Fisher & Paykel FlexiTrunk system |
System Design
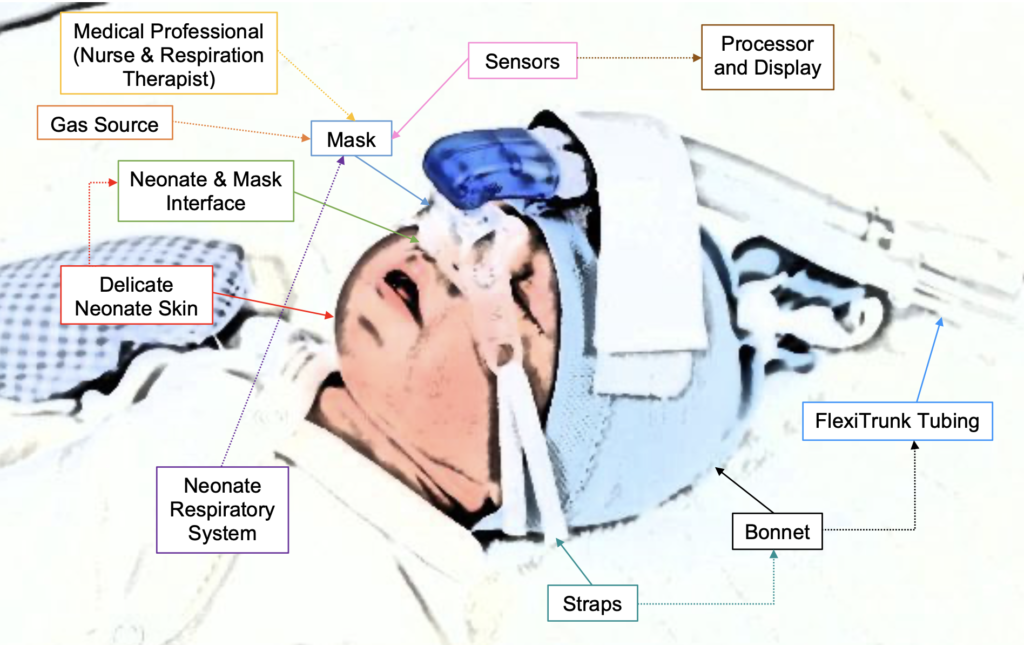
Our design consists of two subsystems that were integrated together to make the final product: the mask subsystem and the sensor subsystem. The mask subsystem is required to deliver CPAP therapy to the infant, and also works to house the pressure sensors that measure the efficacy of the system’s seal. The sensors will not be placed directly on the neonate’s skin, but will be connected to the mask through silicone tubing. The sensors must accurately detect the pressure the mask maintains and then communicate through a circuit to the processing unit. The processing unit will convert the raw measurements obtained by the pressure sensors and will compare the pressures of the main and side chambers to detect differences due to air leaks which will aid in the identification of the area of the mask that has developed a leak. The display then discloses the pressure readings with the hospital’s staff, as well as the location of any identified leaks. In the figure above, a visual representation of the geometric layout of our system shows the different components of both our system and the other systems it interfaces with.
Prototyping Process
Mask Prototyping Process
Our prototyping process to design our mask started with a sketch on a Post-It note. We developed a mask that would have an extra lip on it in order to reduce the overall pressure exerted on the skin and would have sensors attached to it to detect any pressure loss caused by an air leak. From there we took the sketch and developed it into a 3D model using CAD where we worked out more details of the design, such as dimensioning, structural supports, and the attachment to the CPAP machine. A brief overview of the different stages our CAD model went through can be seen below in the figure below.
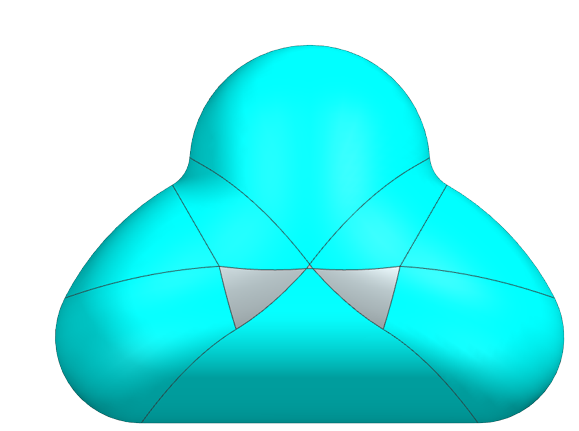
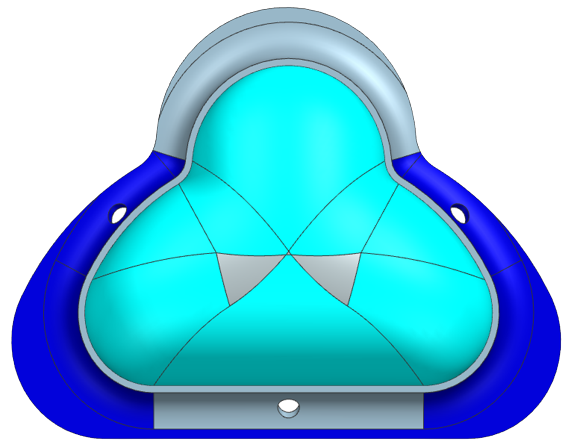
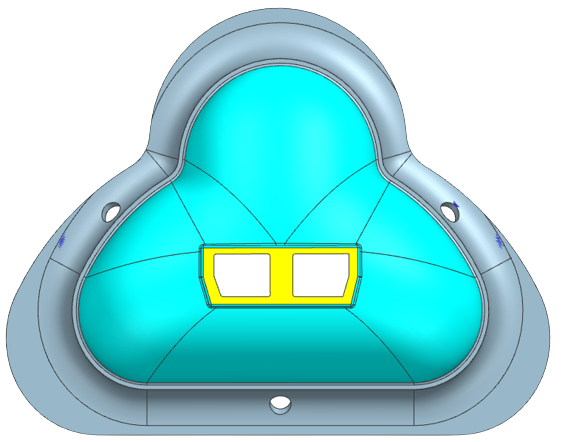
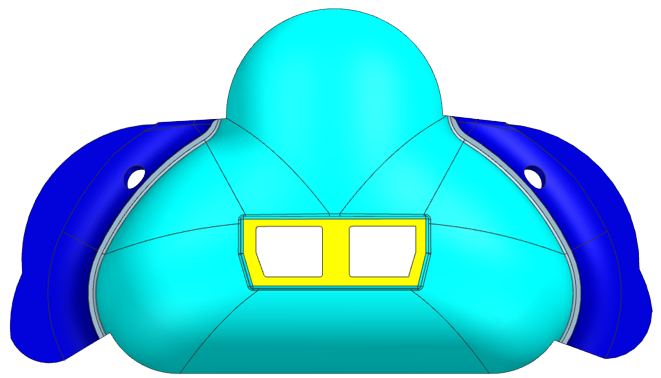
We decided the best way to manufacture our mask was to 3D print a mold and then to use liquid silicone to cast the mask. We chose Smooth-On which has a line for skin-safe liquid silicone with a variety of hardnesses (Smooth-On 2022). We bought four types of silicone Smooth-On: EcoFlex 00-30, EcoFlex 00-50, and DragonSkin 10 NV, and DragonSkin 30.
We then inverted our CAD mask to make a CAD mold. Because a CPAP mask is such an intricate shape, we made a 3 part mold consisting of the bottom, the inside, and the top, as seen below. The mold was 3D printed and hand-finished with dremeling to create contours.
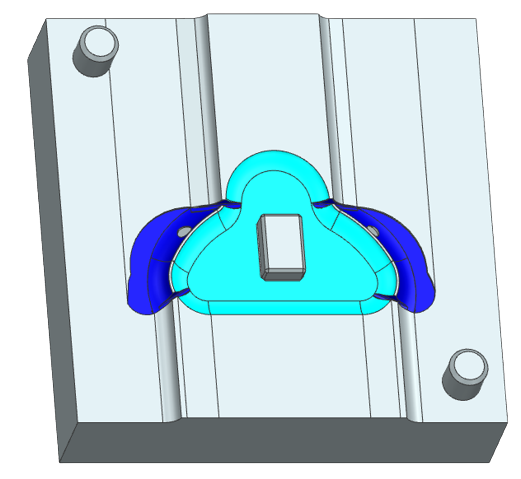
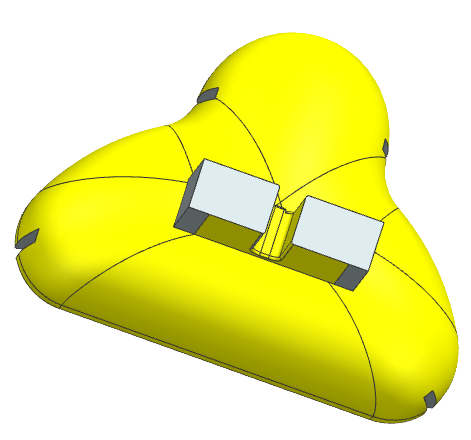
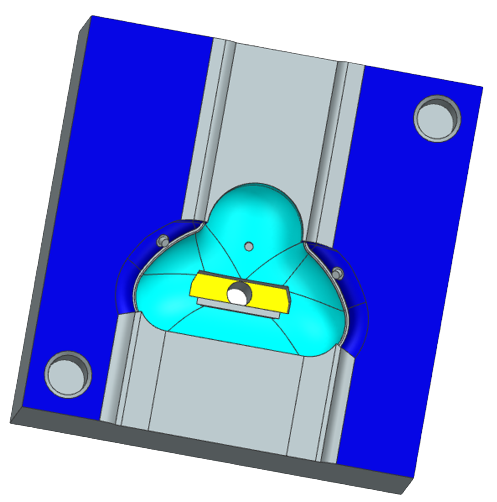
We started with EcoFlex 00-30 to mold our first mask. However, this mask was too soft and did not fully form, so we then tried EcoFlex 00-50. This was also still too soft and malleable, so we switched to DragonSkin 30 which cured into a harder silicone. DragonSkin 30, however, is a more viscous liquid causing large air bubbles to form in the mask which compromises the integrity of it.
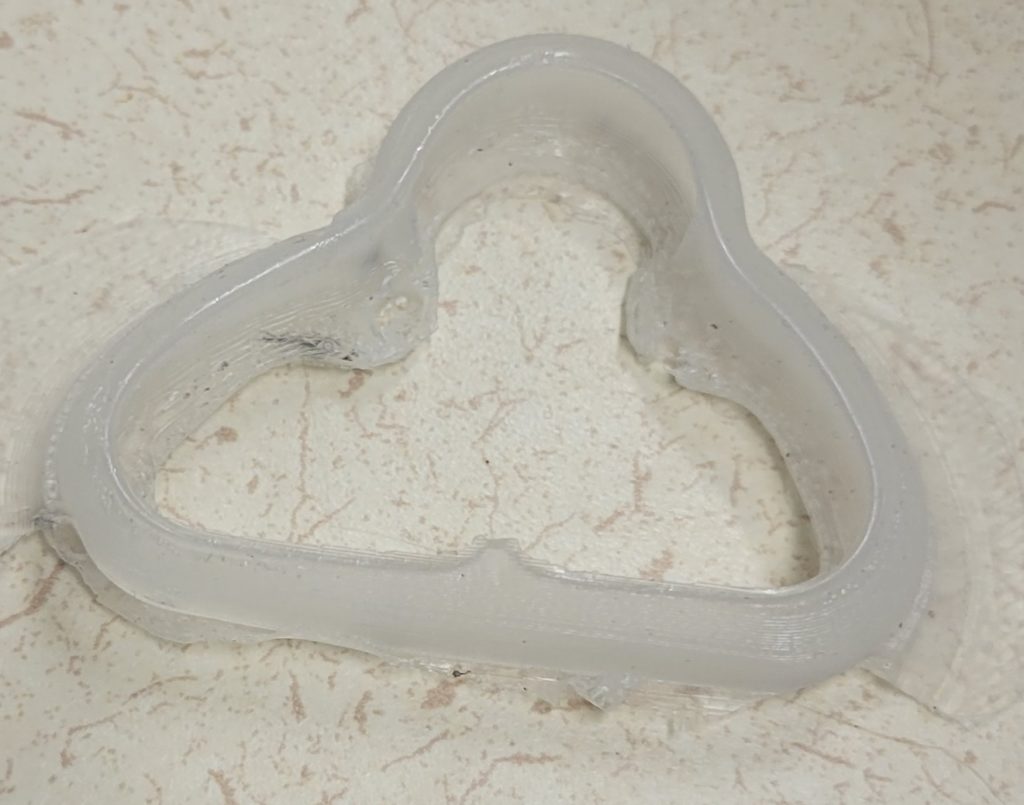
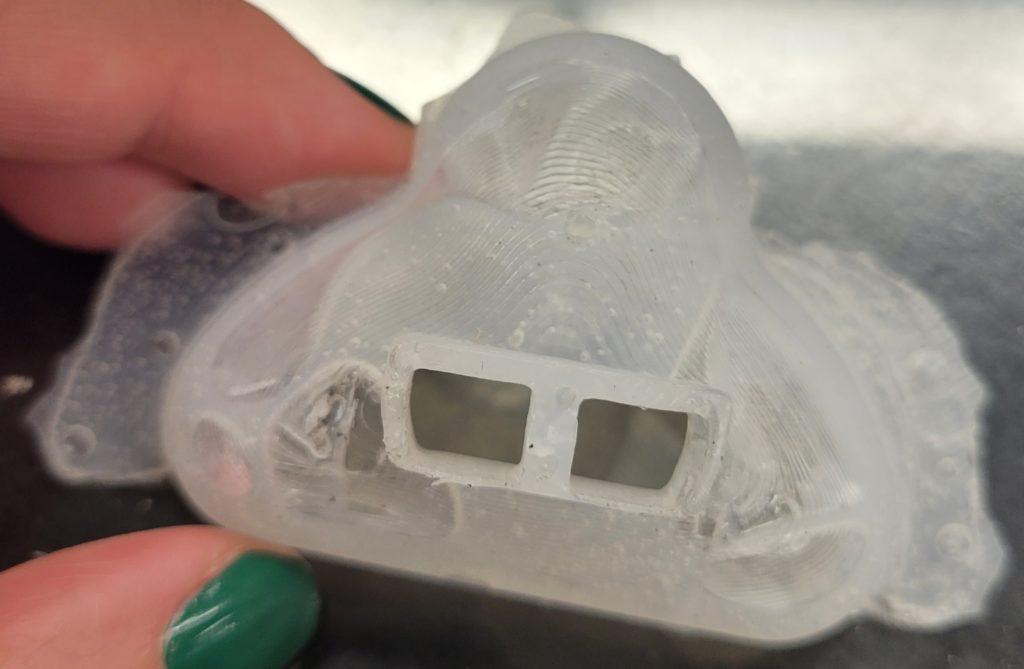
To reduce air bubbles we tried a few different techniques. First, we added silicone thinner to DragonSkin 30 to reduce the viscosity thereby allowing more air to escape. We also used a funnel to pour the Smooth-On into the mold so the added hydrostatic force would reduce air bubbles. Lastly, we made a pseudo-vacuum degasser to reduce air bubbles by connecting a cup to the vacuum nozzle in the Senior Design lab. These techniques greatly reduced the amount of bubbles present in the mask, but there were still some present in our masks. To further reduce the presence of air bubbles, we purchased a new type of Smooth-On, DragonSkin 10 NV, which does not require a vacuum.
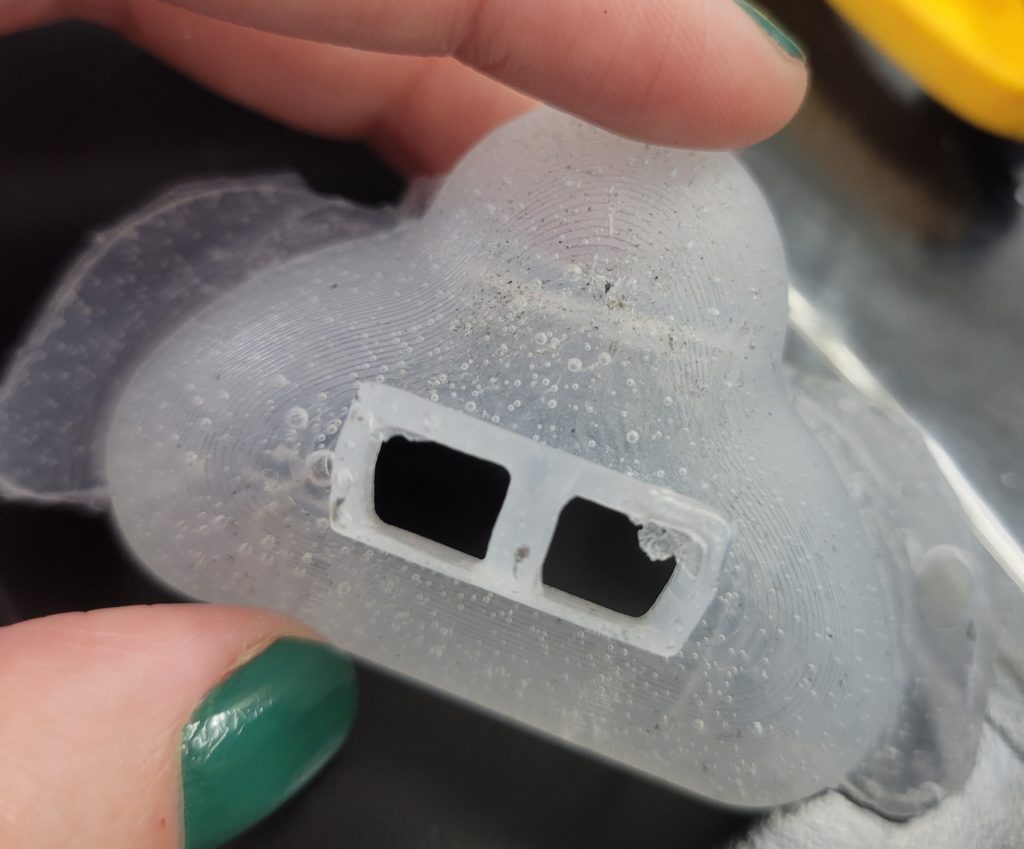
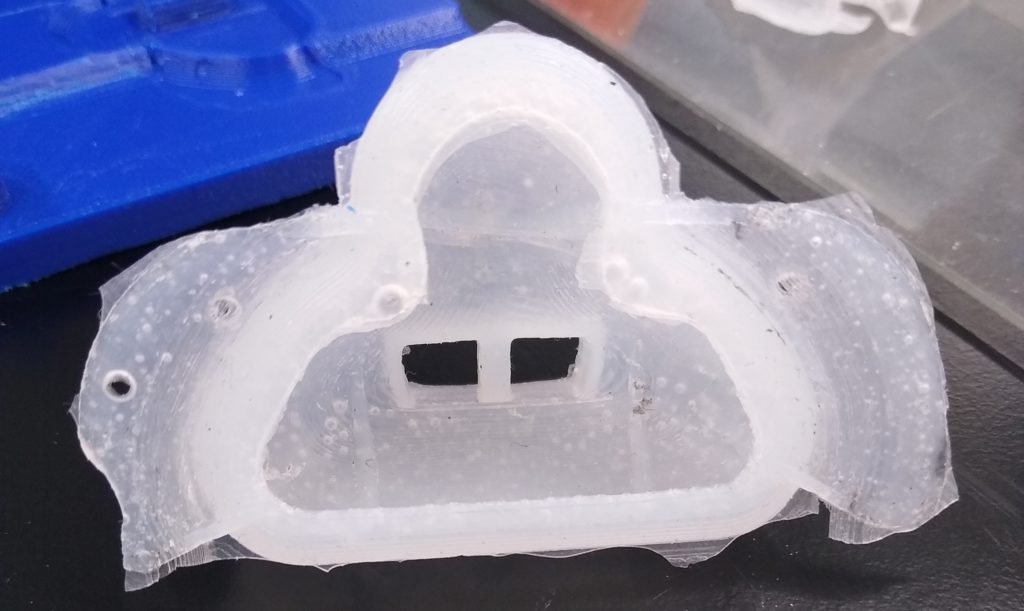
While we were able to reduce the amount of air bubbles, our mask was too large for our testing doll and consequently overlapped with the eyes and mouth of the baby. Since avoiding contact with the eyes of mouth is a design specification, we had to produce a smaller mold and mask. We re-worked the CAD to reduce the size of the mold by 25% and then 3D printed it again.
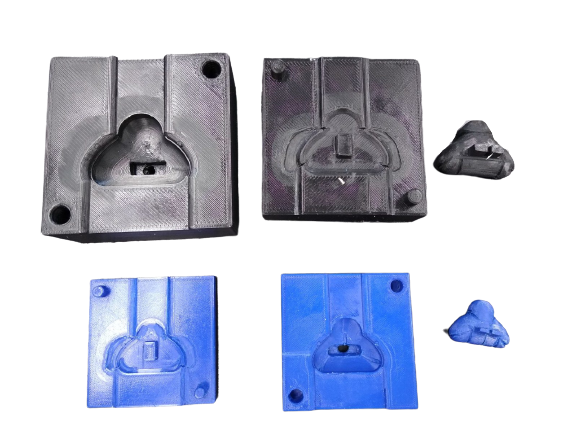
This smaller mask fit our testing doll much better. Between each prototype we also manually filed the mold to make adjustments, such as widening the FlexiTrunk attachment, adding bracing ribs to strengthen the support, and curving the bottom lip to better conform with the neonate’s face.
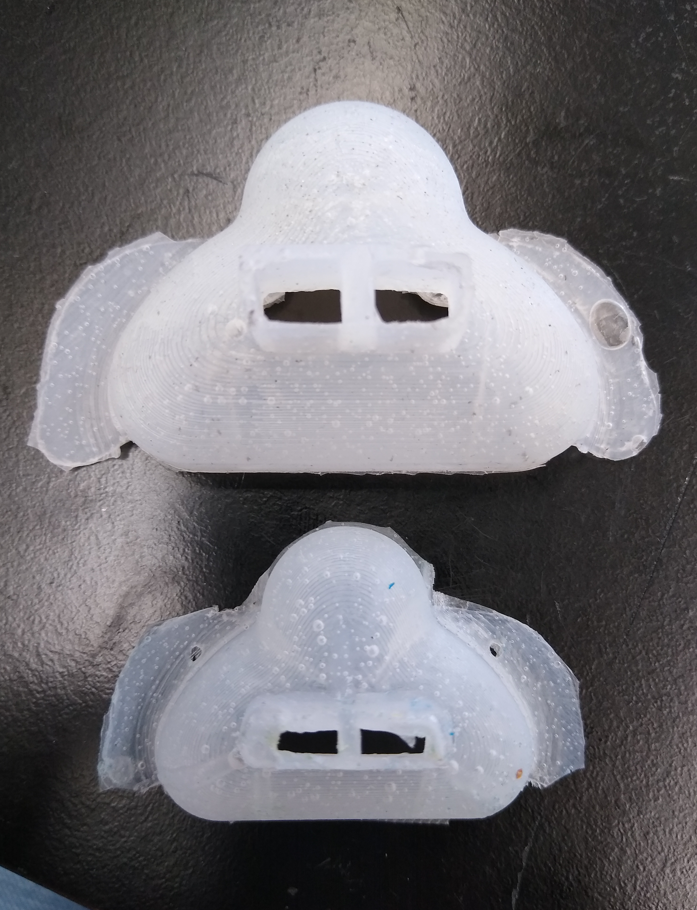
While our masks no longer had air bubbles, they were unable to support the FlexiTrunk attachment. To resolve this, we tried creating a hybrid mask consisting of two Smooth-Ons; the softer DragonSkin 10 NV was used for the bottom of the mask that was in contact with the baby’s face to allow for better conformity, while the harder DragonSkin 30 would be used for the remainder of the mask to support the FlexiTrunk attachment point. During prototyping we used food coloring, yellow for DragonSkin 10 NV and blue for DragonSkin 30, to ensure that the layers of Smooth-On were settling as intended. By combining the DragonSkin10 NV and DragonSkin 30 into a single mask, we were unable to continue vacuum degassing the mask.
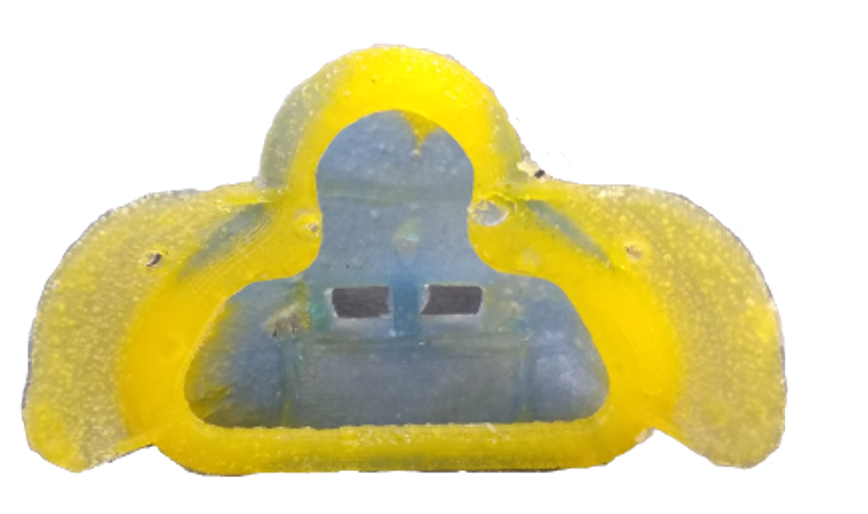
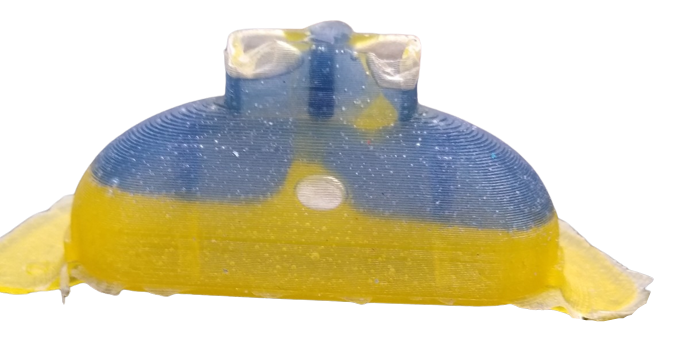
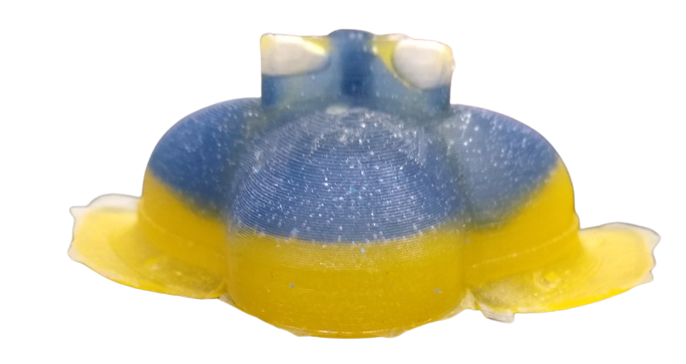
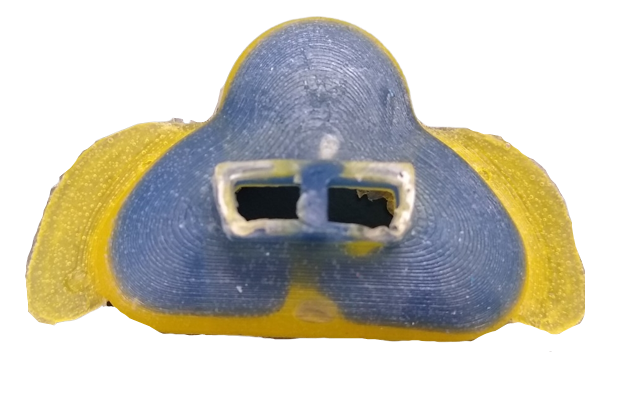
Our final mask is a hybrid mask using our new, smaller mold, but is clear.
Sensor Prototyping Process
A major limitation to the existing CPAP masks is that they are subject to air leaks at the mask-neonate interface with no method of detecting the air pressure delivered at the mask-neonate interface. To combat these issues, we considered incorporating a pressure detection system with sensors. Both electronic and non-electronic detection methods were initially examined; however, electronic pressure sensors were favored due to their ability to offer more accurate readings as opposed to qualitative outputs from the non-electronic methods. Within the category of electronic pressure sensors, our original choice was to utilize surface contact pressure sensors that would be placed directly at the mask-neonate interface to measure the pressure exerted by the mask at the specific location. Upon further deliberation, it was decided that the placement of these sensors on the neonate’s delicate skin could pose deleterious consequences due to biocompatibility and safety issues. Additionally, the sensors could also contribute to the development of pressure injuries at the location of the sensors. To mitigate these issues, we decided to employ gas pressure sensors. Gas pressure sensors would not have to be placed on the skin to be able to measure the pressure. Instead, these sensors can be connected with tubing to the area of interest to obtain pressure readings. We opted to use the Honeywell ABP2DANT001PGSA3SN gas pressure sensor; this particular sensor has been approved for medical usage for CPAP and has a range of 0-1 psi making it highly accurate.
The sensors are connected with silicone tubing to holes on the right and left sides of the mask and connected to an Arduino Uno processor to convert the measurements to easily readable data. The processing unit will display the pressure delivered to the neonate in the main central chamber on an LCD screen and will light up the corresponding LED to notify whether the right or left side of the mask has developed an air leak. To test the functionality of our system, a pseudo-CPAP system was created by connecting an air source to the FlexiTrunk attachment and mask with tubing to deliver air to our testing doll, the Newborn Anne doll. The outlet to the FlexiTrunk attachment was extended with more tubing to a column of water to measure the input pressure in centimeters of water. With this system, we compared the pressure measurements from the pressure sensor attached to the main chamber of the mask to the amount of pressure determined by the column of water in centimeters. Additionally, to determine the effectiveness of our leak detection system, we introduced a leak to the right side, to the left side, and to both sides, and then analyzed the ability of our system to determine the occurrence and location of these leaks accurately.
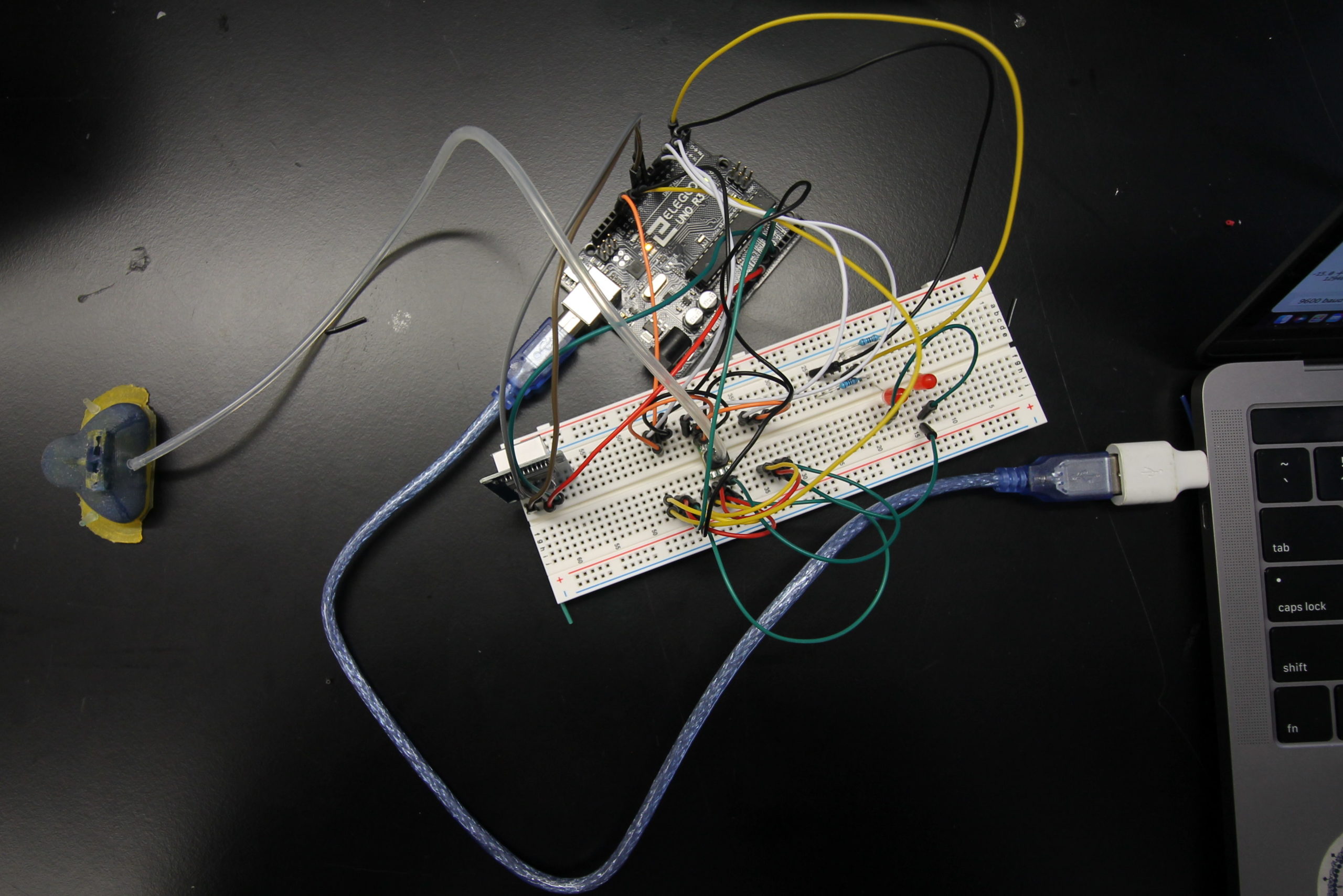
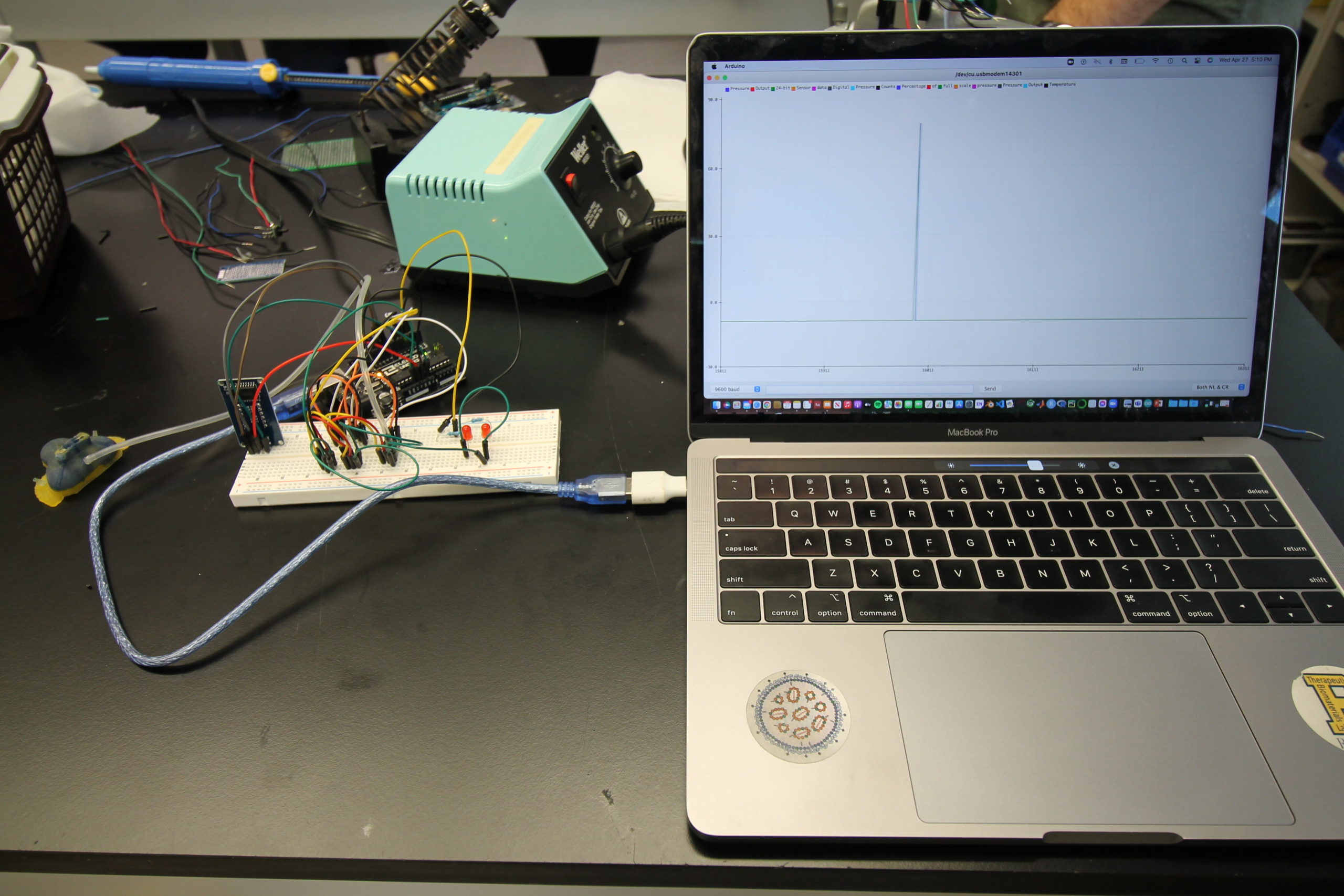
Testing
For our testing, we used a Newborn Anne doll loaned to us by Dr. Dylag from the NICU. This doll is an anatomically correct CPR training doll that has inflatable lungs so we could simulate delivering CPAP. However, because the doll is anatomically correct, the mouth and nose are connected. Thus, when air is delivered to the nose, it would come out of the mouth instead of being delivered to the lungs. To solve this issue we taped the mouth shut, as shown below. We also designed a pseudo Bubble CPAP and incubator chamber for our testing. Our pseudo Bubble CPAP machine was created by connecting an inlet tube to an air supply in the Senior Design lab that fed into the FlexiTrunk and our mask. The outlet to the FlexiTrunk was attached with a separate tube to a column of water that would measure the amount of pressure being delivered in centimeters of water. We also dremeled a hole into a plastic storage box to simulate the incubator that a neonate would be in the NICU where the FlexiTrunk attachment rests on the opening in the incubator.
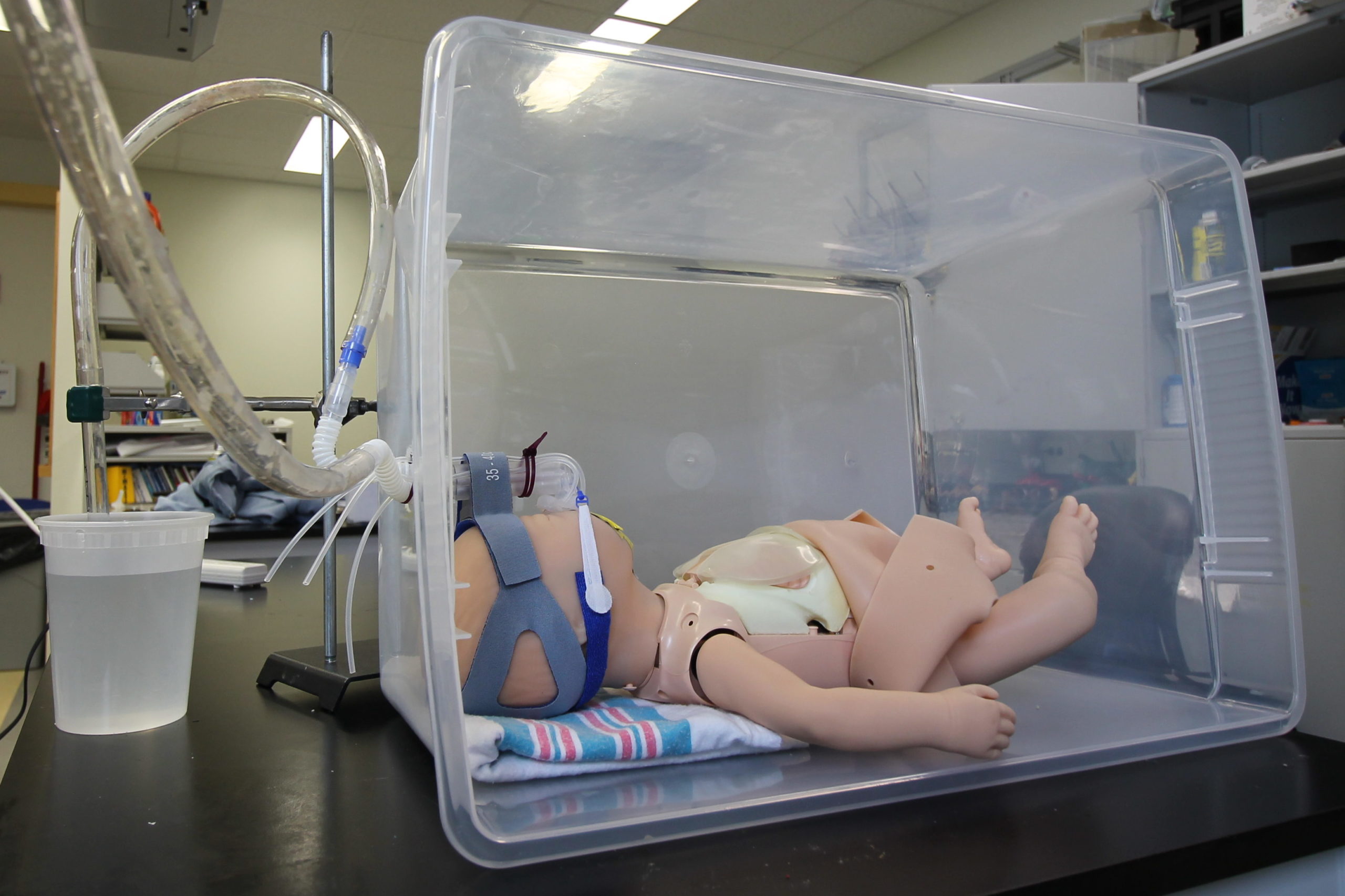
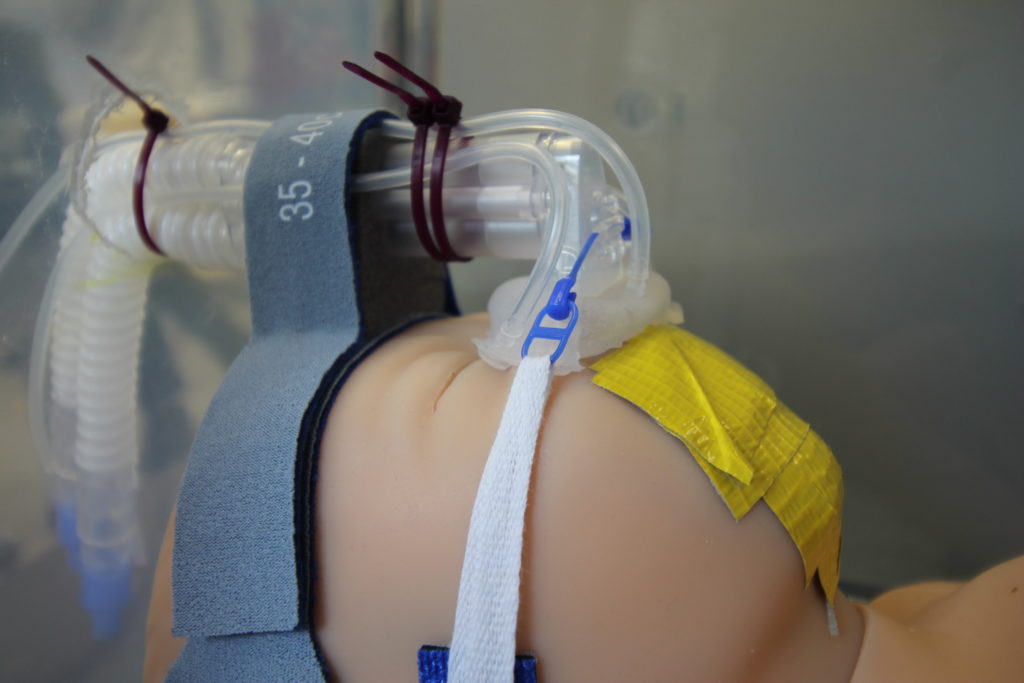
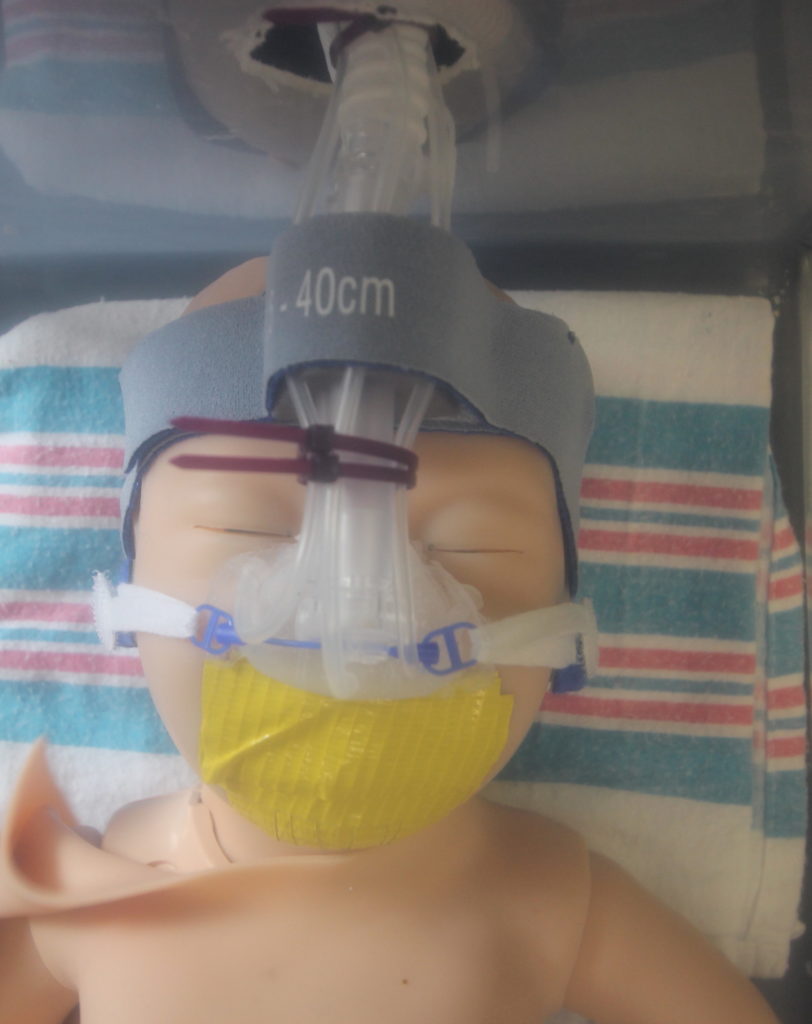
Pressure Reduction Testing
One of the main concerns with CPAP masks is the occurrence of pressure ulcers on the neonate’s face which is why our second most important customer need was to reduce the pressure exerted on the skin. We performed this test to see if our mask exerts less pressure than the current Fisher & Paykel mask by comparing the contact area of our mask to the Fisher & Paykel mask when the same force is applied. Since pressure is force divided by area, increasing the area will decrease the pressure.
The contact area of the mask was found by placing a Post-It note on a curved surface and then placing the mask on it and applying a predetermined force. The image was then uploaded to ImageJ where the area could be found. The contact area of our mask was compared to the contact area of a similar-sized Fisher & Paykel mask and one of our masks with the wings cut off. Our mask had an area 90.25% greater than the Fisher & Paykel mask and had an area 37.80% greater than our mask without wings meaning that the wings on our mask significantly reduce the pressure on a neonate’s face.
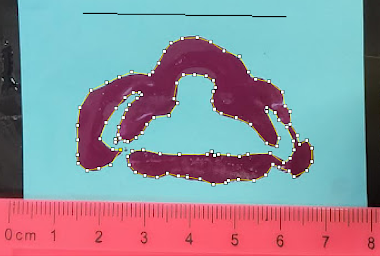
Stability of Mask on Face
This test was used to evaluate whether or not our mask system is prone to slippage on the neonate’s face when compared to current mask models. Babies move and their equipment can be bumped, so it is important that the mask can stay on when movement is occurring. Our most crucial customer need is to maintain pressure. However, when the mask slips, an air leak forms. This test was also used to address our fifth need which ensure that the mask does not overlap with the eyes and nose by observing if the mask has a tendency to shift into the eyes or nose with movement.
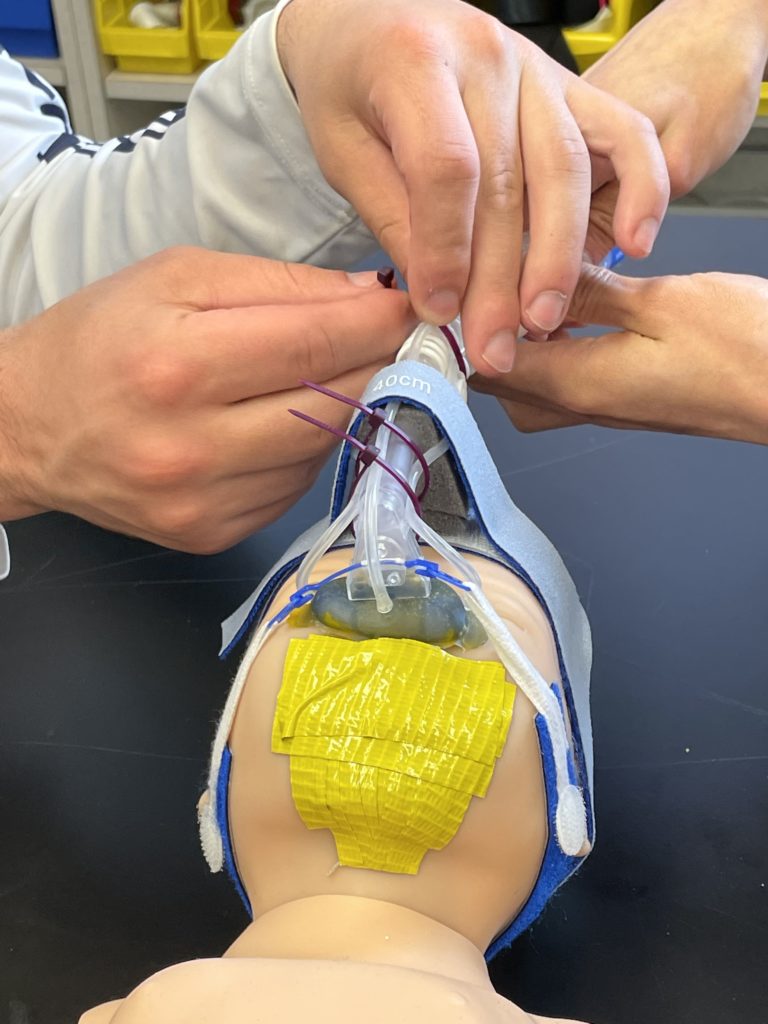
We found that our mask only slipped one more time than the control did, and this occurred when we pushed the FlexiTrunk to the left. Otherwise, both our mask and the Fisher & Paykel masks slipped when the same movement was applied. Neither mask slipped when the masks were pushed to the right, when the head was twisted with the FlexiTrunk fixed in place, or when the baby was gently shaken with the FlexiTrunk and tubing hanging loose. Both masks did experience slippage when the FlexiTrunk was pulled or pushed, the baby moved onto its side, or when the baby was shaken with the FlexiTrunk tubing fixed in place. While our mask did not solve any slippage problems, it did not cause significantly more slippage problems. The Fisher & Paykel mask slipped 44% of the time while our mask slipped 48% of the time.
Ease of Use
The test was performed eight times by four different group members. Each group member started with a mask on and was timed to determine the length of time it would take them to remove the mask and connect a new mask (of the same type) on. For example, a Fisher & Paykel mask would be removed and replaced with another Fisher & Paykel mask, or our mask would be removed and replaced with another one of our masks. Both the Fisher & Paykel mask and our mask were tested. We also switched which mask was being tested first to account for any learning curves.
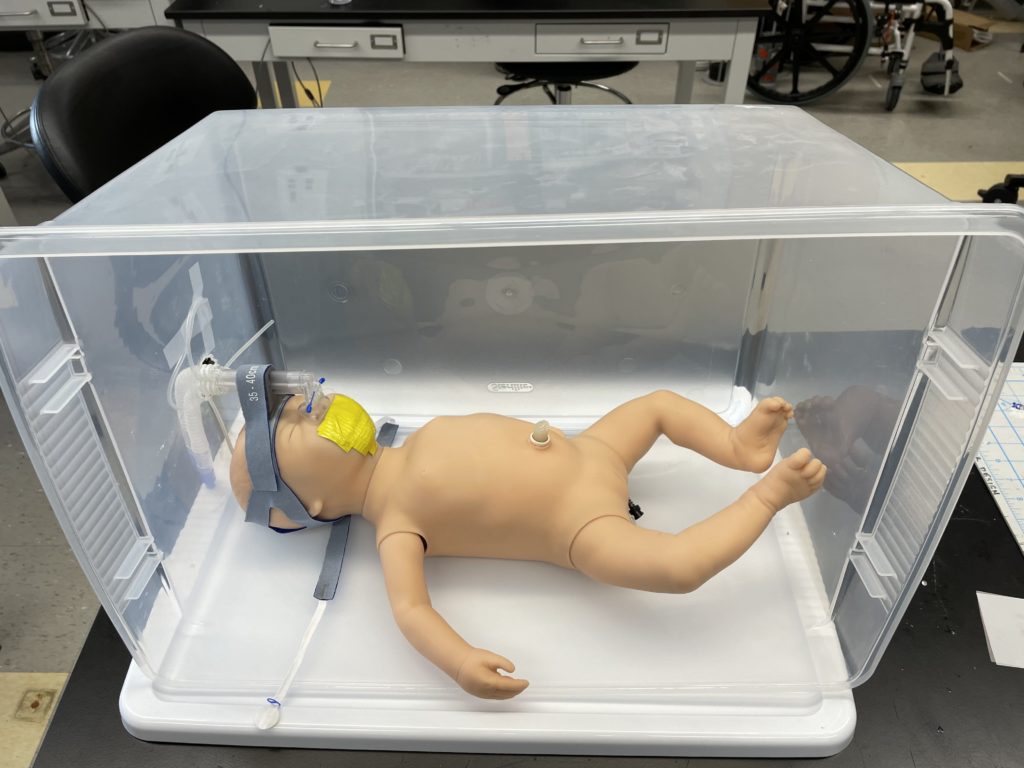
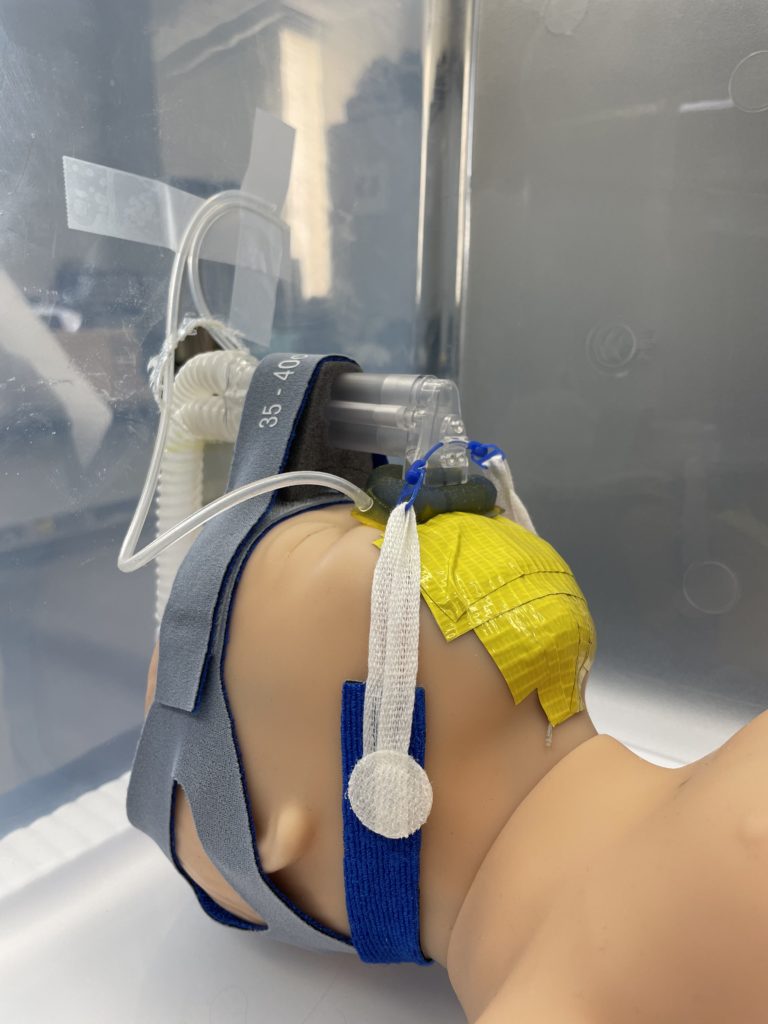
On average, it took 18.29 seconds longer to put on our mask with the sensor tubing than the control Fisher & Paykel mask. It should also be noted that people were faster on their second time putting on the mask, showing that after some training this time could be reduced.
Next Steps
While our current prototype features many advantageous design prospects, limitations in budget and access to materials has impacted our ability to design the ideal mask. For future steps, it would be ideal to print our prototype with medical grade silicone that is used for current neonatal CPAP masks. This would ensure biocompatibility and avoid safety concerns since this material is already FDA approved. Additionally, by using medical grade silicone, the material properties of the mask would be similar to the current models. While our hybrid masks made of DragonSkin 10 NV and DragonSkin 30 try to mimic the hardness of the Fisher & Paykel masks by using silicone rubber with Shore 10A and and Shore 30A respectively, the hardness is not identical. Using the same hardness will help to guarantee that the weight of the FlexiTrunk attachment is supported and that the mask will not compress.
Improvements could also be made to the manufacturing process of the masks itself. Due to our limited budget and the high cost of 3D printing silicone, our manufacturing process involved multiple steps. With our current process, a mold was created on CAD, 3D printed, and then injection molded with Smooth-On. This process, however, is not ideal since variations in the mask can occur due to difficulties with the removal of excess silicone. To mitigate variations of the mask, 3D printing the mask would be ideal.
Our mask also utilizes plastic adapters to connect the mask and sensors together with silicone tubing. Plastic, however, is not safe to be in contact with the neonate’s skin and poses a safety issue. In addition, adding the plastic adapters could contribute to the occurrence of pressure injuries at these locations and cause skin irritation. To resolve this, it would be beneficial to add connection points to the mask design itself so it could be 3D printed in silicone. Due to the complicated nature of creating a mold and injection molding, our design was unable to incorporate a connection point into the CAD model, so plastic adapters were used instead.
Lastly, with our sensor subsystem, improvements can be made to decrease the size of the circuitry and create a more economical display. Currently, the pressure measurements read by the sensors are processed by an Arduino Uno. While this processor was affordable and offered a helpful inventory of example codes to help code our processors, the efficiency can be improved and size of the processor could be reduced by purchasing a more expensive option.
Constraints
Regulatory
Before our neonatal CPAP mask could go to market, it would have to be cleared by the FDA. There are two possible pathways to market for our CPAP mask, either a 510(k) using multiple predicates or a De Novo Classification Request. When combined The Infant Flow NCPAP Mask from SensorMedics (K984254) and the NAPA LP-15 Airway Pressure Monitor (K172284) cover most aspects of our neonatal CPAP mask with sensors, however there are some parts (such as the double lipped design) that are different and might raise questions of safety and efficacy for the FDA, which means the 510(k) application might not be accepted. The other option would be to go through the De Novo Classification Request and claim that there are no legally marketed predicate devices for our mask. However, this process is ten times more expensive than the 510(k), so would be less desirable (FDA 2021a).
To best decide which regulatory pathway to utilize, it would be best to submit a Pre-Submission to the FDA, which is a free process where we could ask the FDA questions about our application and receive written feedback (FDA 2021b). We could ask them, “Does the FDA have concerns over us using the Infant Flow NCPAP Mask from SensorMedics, K984254, as well as NAPA LP-15 Airway Pressure Monitor, K172284, as multiple predicate devices given that the Senior Design Neonatal CPAP Mask combines a neonatal CPAP mask with an airway pressure monitoring system?” Then, from the feedback we receive, we could either submit a 510(k) with multiple predicates, or a De Novo Classification Request. From there we would receive FDA clearance and could market our neonatal CPAP mask.
Manufacturing
To further the production of masks, we would ideally look to use advanced manufacturing processes that would allow us to use different types of silicone that would not only be better at supporting the FlexiTrunk attachment, but would also be medically safe to put on the face of neonates. To start this process, we would take 3D scans of our current molds to get digital copies of the mold that can be sent to Online manufacturers who can then make a permanent and ideal mold made out of Stainless Steel 304, which is what many medical instruments are made of. Our mold would either be made on a CNC, or possibly through new and emerging technology such as Metal Injection Molding. Once we get a finished mold, we can begin making masks. Currently our mask is limited to 30A hardness simply because higher hardness silicones have much higher viscosities, which tend to create large air bubbles in our mask. Injection molding and vacuum degassing would allow us to use harder silicone, which would better support the FlexiTrunk. Injection molding would ensure that complete and even distribution of silicone would be achieved, while vacuum degassing would vacate air bubbles left in the silicone from injection. A combination of these would give us an ideal product.
Ethics
Ethical conflicts may arise in the choice as to whether or not our mask will be the mask used for a given neonate’s CPAP therapy. Choosing either use or avoidance of our mask introduces ethical dilemmas in the perceived and actual qualities of care provided, as provision of an additional choice may lead to excess stress and harm that outweighs the benefits. The ease of checking the quality of CPAP therapy with our mask also risks encouraging complacency in care that could lead to unanticipated negative side effects. In addition, our sensors may lead to increased modifications of medical care that may have unintended negative effects on neonates, especially in the risk of allowing untrained individuals to notice leak development and attempt to modify our mask.. The ethical dilemmas surrounding neonatal care are complex, and the downstream effects of choices may lead to unintended consequences that must be weighed against the expected benefits selected for.
Economic
Ideally, our mask could reduce the frequency of checks needed by nurses, and perhaps units with less staff could also be making steps towards incorporating CPAP into their facilities. However, even if the frequency of checks is reduced, there are other additional costs due to the delicate 3D printed features, sensors, and displays of our new design concept that will increase the overall cost per mask and thus limit the affordability of the mask. Additionally, all of this is under the assumption that a hospital was already able to afford a respiratory machine as expensive as CPAP. This means that, despite the benefits of our new mask features, increased costs might be limiting the number of new hospitals that can afford it.
Global
Our improved CPAP mask is specifically designed for the Fisher & Paykel CPAP device. As a result, this mask is not compatible with other CPAP devices manufactured by different companies. Additionally, this mask is not compatible with CPAP devices used in low income countries. It is important that future advancements in the production of this mask take these incompatibilities and limitations into consideration in order to make this mask more accessible globally and improve the delivery of CPAP therapy to the neonates worldwide.
Social
Our design will primarily impact prematurely born newborns who require CPAP to support their breathing. These babies will grow and develop, so our mask will be available in three sizes to accommodate these changes. These sizes are modeled after the sizes of the current CPAP masks used in the NICU. Additionally, this mask was designed to avoid covering the infant’s eyes and mouth to avoid irritation and for feeding tubes. However, neonates with craniofacial abnormalities, particularly cleft lip and/or palate, cannot use this mask until they have corrective surgery to bridge this gap since an air leak would be present making CPAP ineffective. Overall, this mask will function like the current models used and will aid in the development and survival of the neonates. Neonates who do undergo CPAP are eventually discharged from the hospital, but are at a higher risk of developing medical problems due to underdevelopment at birth, so their quality of life might differ from full-term babies. Additionally, it can be considered unethical to administer CPAP therapy to neonates with a low survival rate since it would cause them nasal and facial pain. Neonates are not autonomous and are unable to verbalize their wishes and pain, so their treatment is dependent on their parent’s decision.
Our design also has the potential to allow for greater parental interactions with the neonate. From our visit to the NICU, we learned that parents were afraid to pick up their child for fear of causing the mask to shift from the baby’s face which can decrease the amount of air the neonate is receiving and increase the likelihood of pressure injuries. With our design, ease of detecting air leaks and an increased neonate-mask interface contact area should remedy both of these issues helping to ease the worry that parents feel.
Our Team
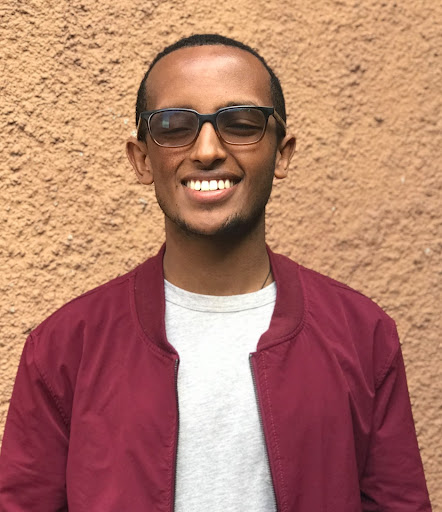
Cell and Tissue
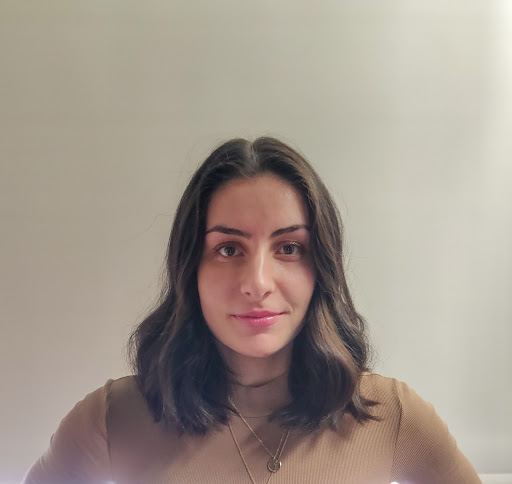
Cell and Tissue
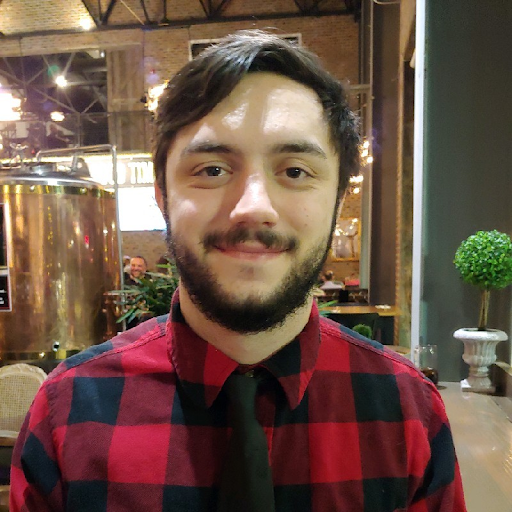
Biomechanics
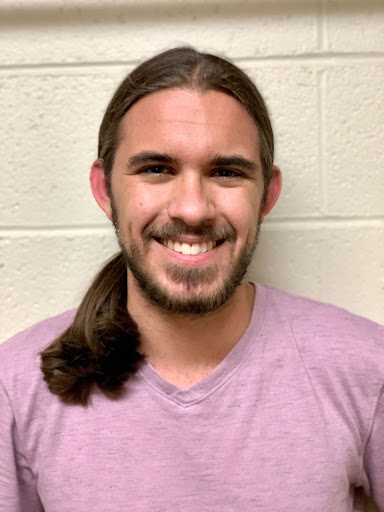
Cell and Tissue
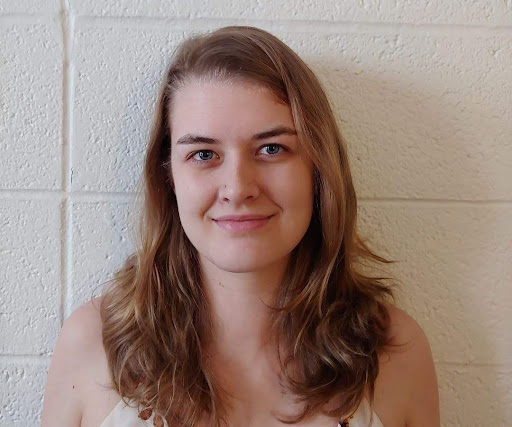
Biomechanics
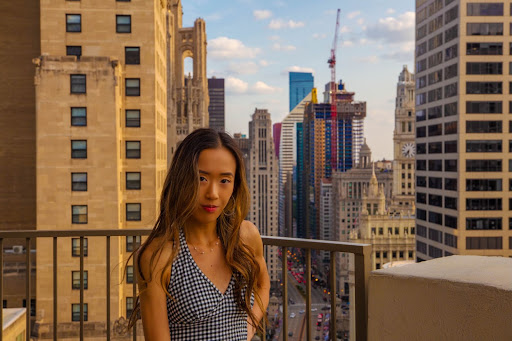
Cell and Tissue
Our Team in the Lab!
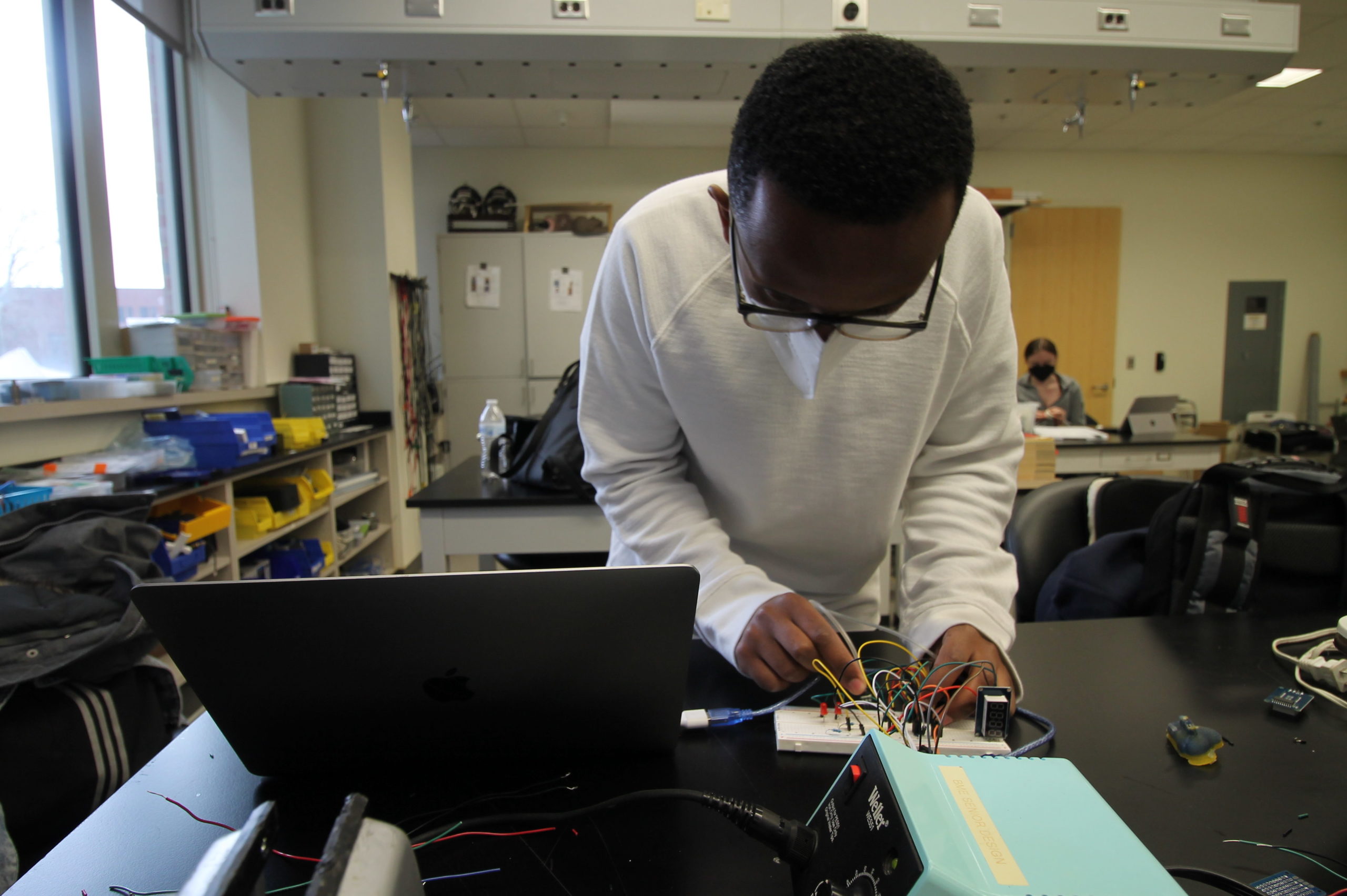
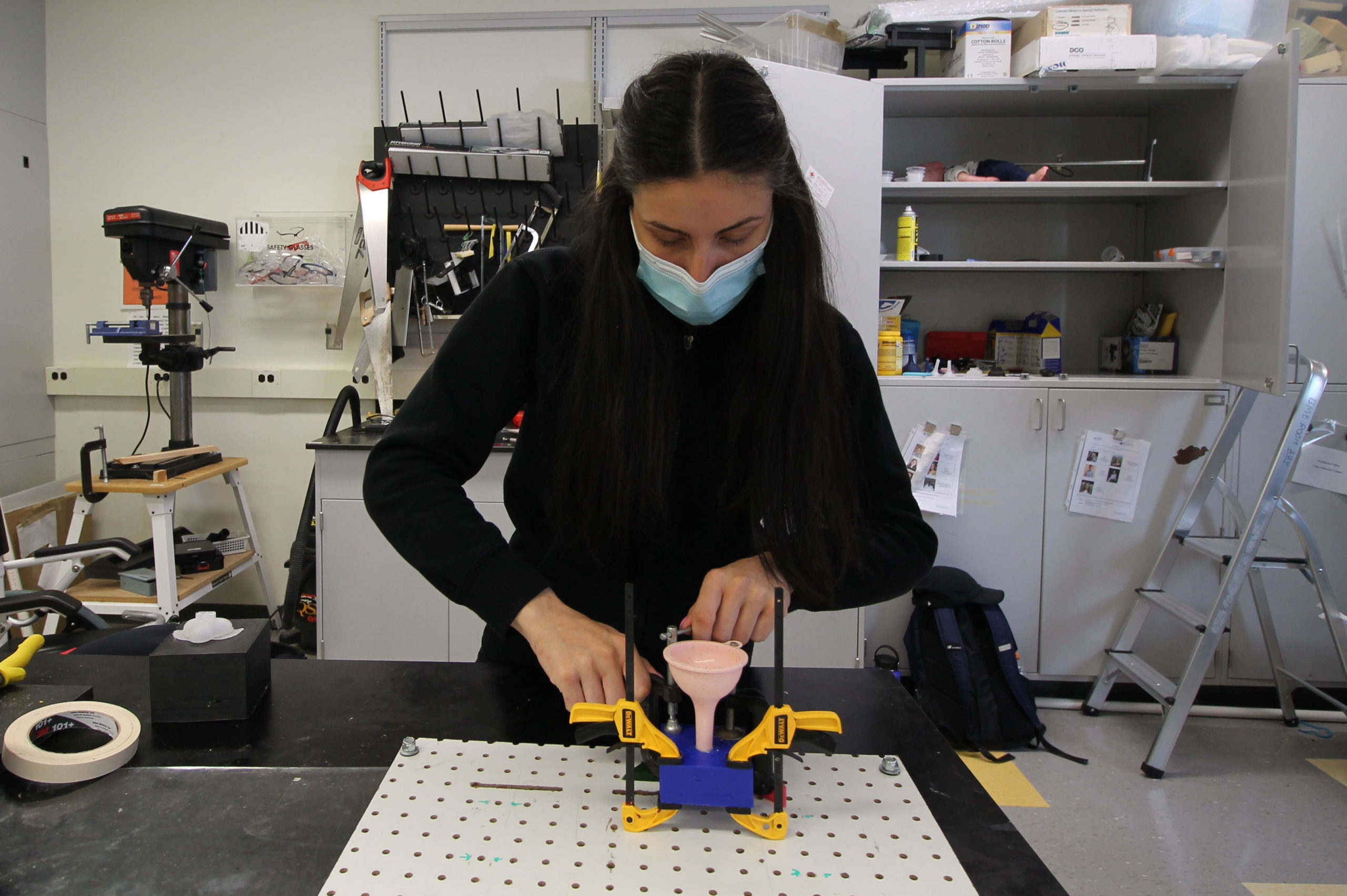
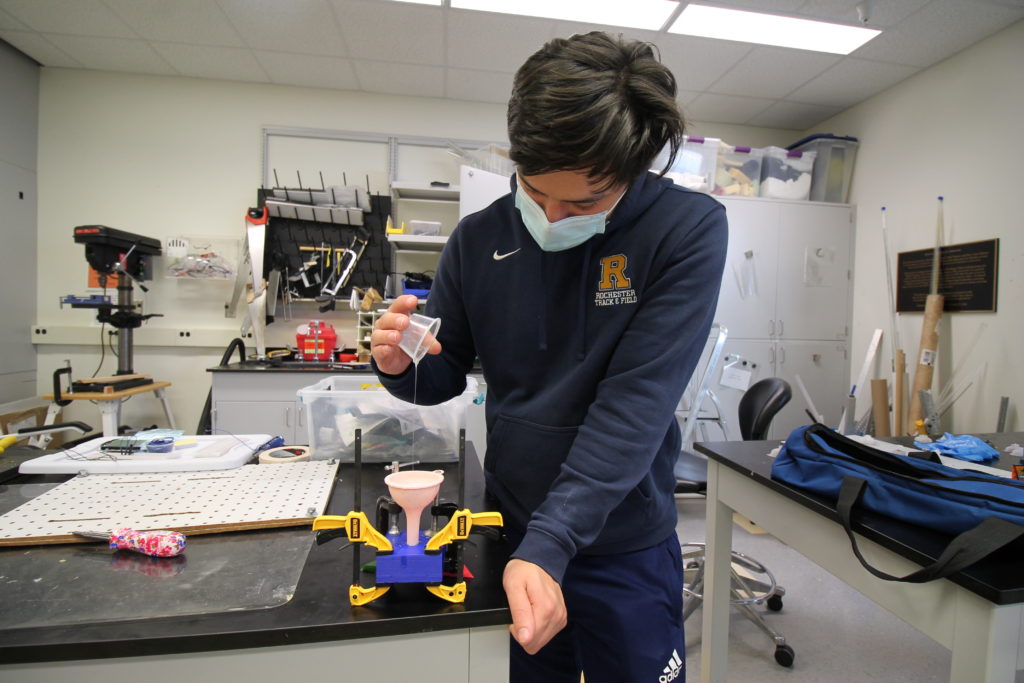
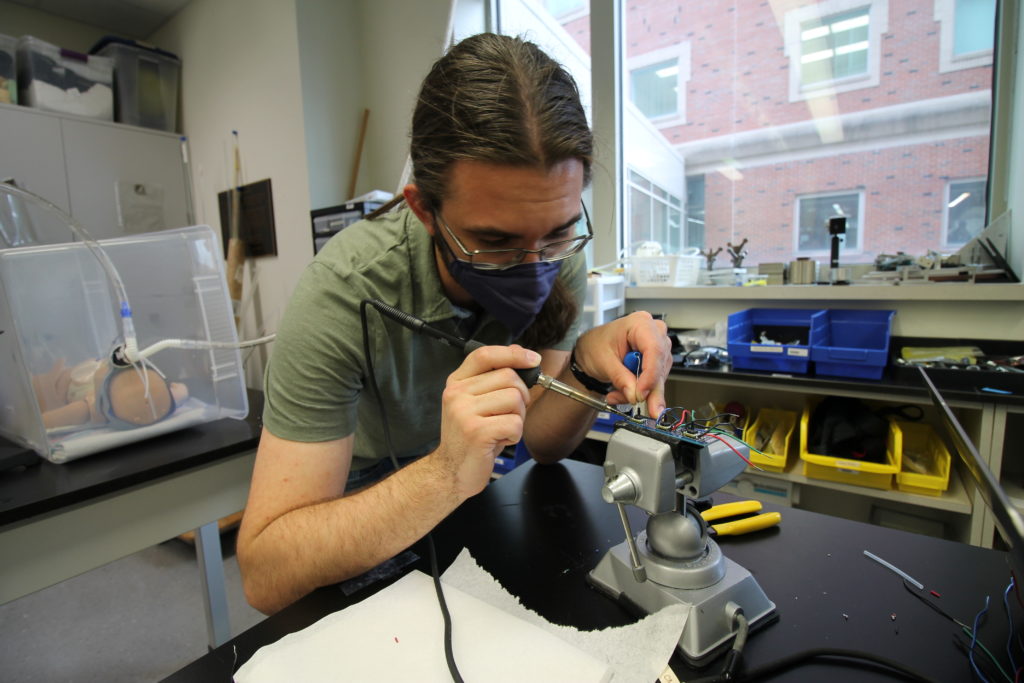
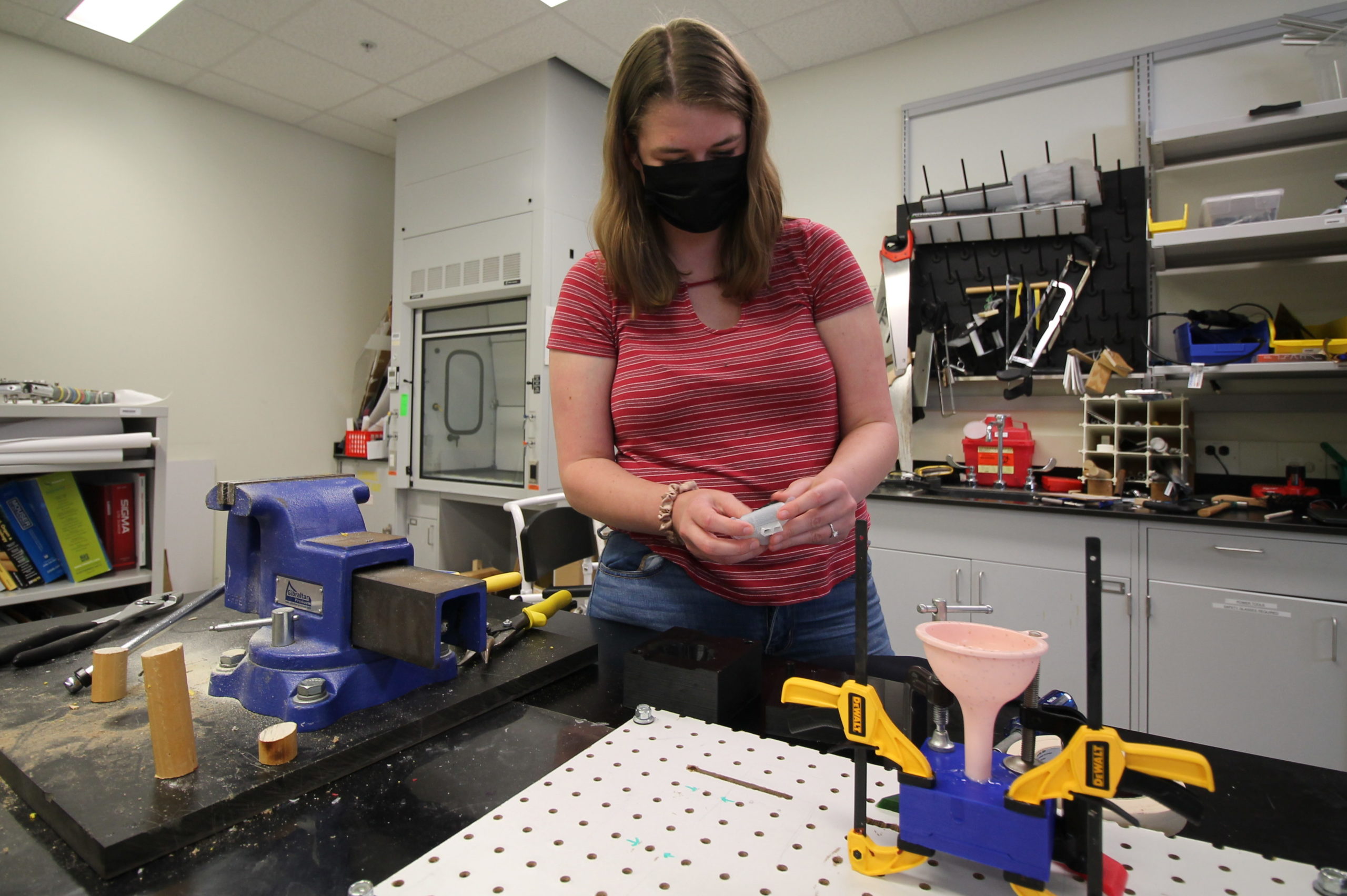
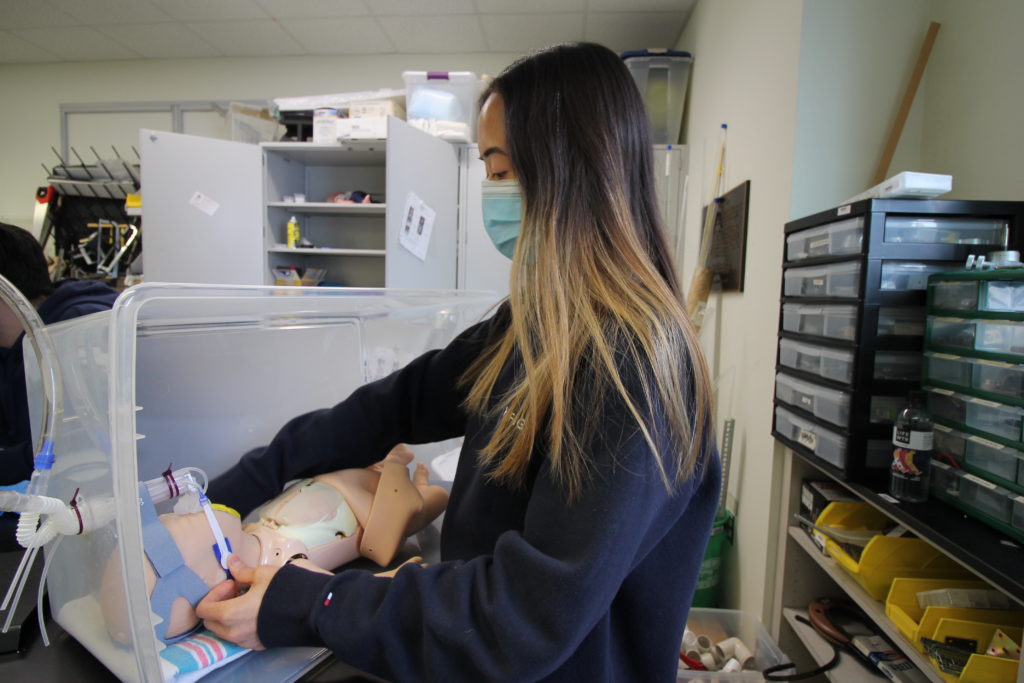
Customer
Dr. Andrew Dylag
Neonatologist, URMC
Supervisor
Dr. Anne Luebke
Department of Biomedical Engineering, University of Rochester
Project Liaison
Allison Coon
CMTI, University of Rochester
Senior Design Professor
Dr. Amy Lerner
Department of Biomedical Engineering, University of Rochester
Senior Design Professor
Dr. Scott Seidman
Department of Biomedical Engineering, University of Rochester
Acknowledgments
Thank you to Dr. Dylag for approaching us with this problem, providing us with the opportunity to visit and learn about the usage of CPAP in the NICU at Golisano Children’s Hospital, meeting with us to discuss our ideas, and for providing insightful feedback and advice for our design and testing plans.
Thank you to our supervisor, Dr. Anne Luebke and our Project Liaison, Allison Coon, for meeting with us every week to discuss our project and provide thoughtful feedback on our design.
Thank you to Dr. Lerner and Dr. Seidman for teaching Senior Design, providing the opportunity for our team to work on this project, and for your continuous support throughout the year on our project.
Thank you to everyone else for helping us with our project, including: Martin Gira, Theo Couderc, Mahllet Beyene, the staff of the NICU, the family at the NICU we visited, and Christine Pratt.
References
Dylag, A. 2021, NICU Visit, November 5.
FDA (2021a) Medical Device User Fee Amendments (MDUFA) [Online]. Available at: https://www.fda.gov/industry/fda-user-fee-programs/medical-device-user-fee-amendments-mdufa
FDA (2021b) Requests for Feedback and Meetings for Medical Device Submissions: The Q-Submission Program – Guidance for Industry and Food and Drug Administration Staff [Online]. Available at: https://www.fda.gov/media/114034/download
NORD (National Organization for Rare Disorders) 2021. Respiratory Distress Syndrome, Infant. [Online] Available at: https://rarediseases.org/rare-diseases/respiratory-distress-syndrome-infant/
Smooth-On (2022) Silicone Rubber – Platinum Cure [online]. Available at: https://www.smooth-on.com/category/platinum-silicone/