Pediatric Surgical Group
Pediatric Expansive Hernia Repair – A CDH Treatment
Abdullah Abuomar, Marianna Aviles Jubis, John Moyer, & Terra Spurgeon
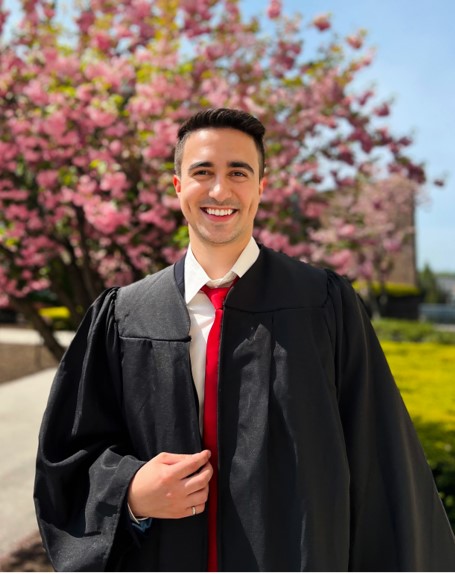
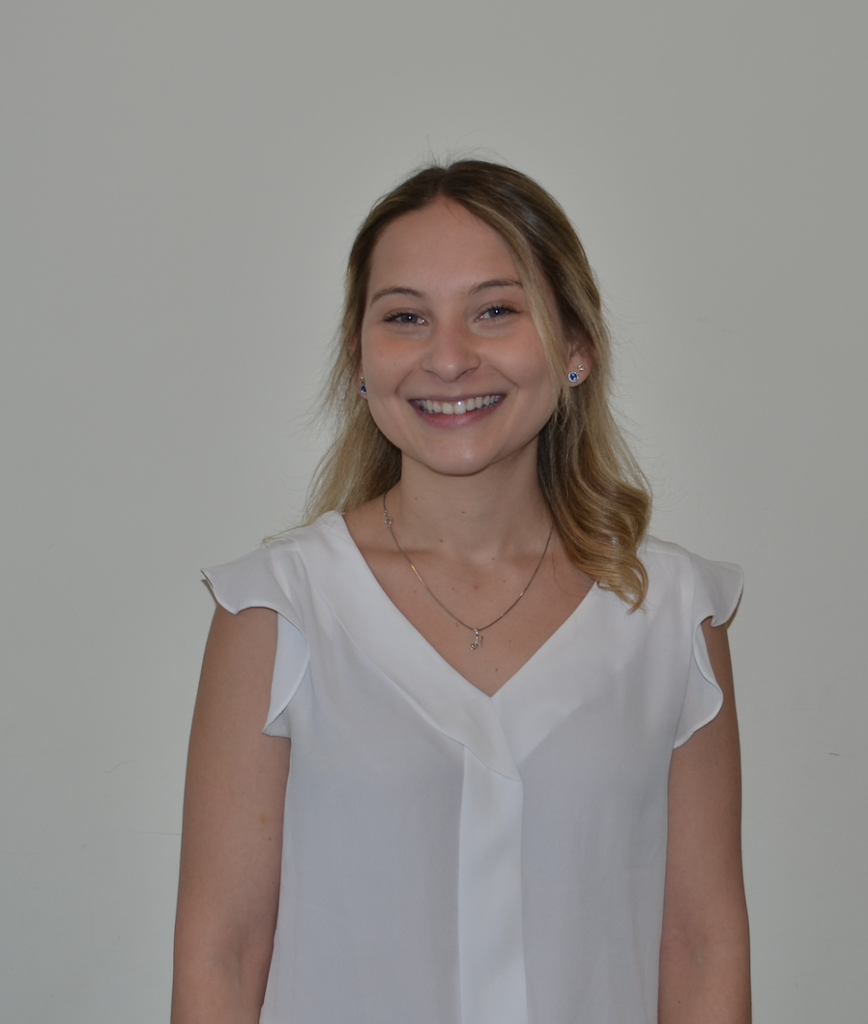
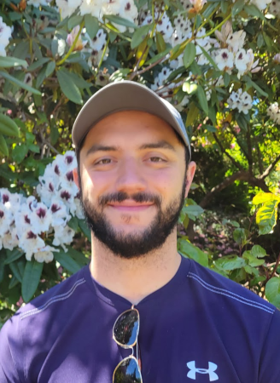
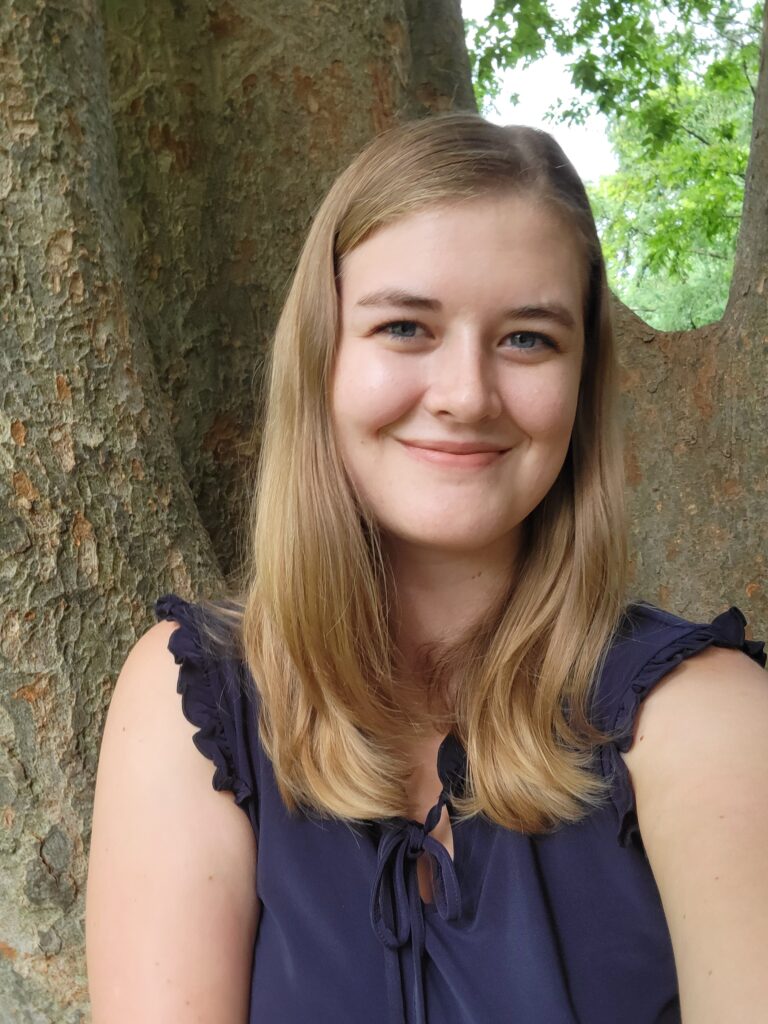
Table of Contents
Disease State Fundamentals – CDH
Limitations and Future Considerations
Biodesign Need Statement
A way to repair a severe congenital diaphragmatic hernia (CDH) in pediatric patients to reduce the rate of postoperative diaphragmatic re-herniation.
Disease State Fundamentals – CDH
A congenital diaphragmatic hernia (CDH) is a defect where an infant has a malformation in their diaphragm, forcing the organs in the abdominal cavity into the chest cavity, as seen in Figure 1 below. The pleuroperitoneal canal is meant to close between the 6 to 8th week of gestation; however, failure to do so results in a congenital anomaly [1]. According to the CDC, about 1 in every 3000 babies is born with this disease [2]. When the abdominal organs are pushed into the thoracic cavities, the baby’s lungs cannot fully develop, leading to potential respiratory failure and death. The average survival rate for CDH is between 70-80%, however the survival rate for severe cases where a patch is used is only 15-25% [3].
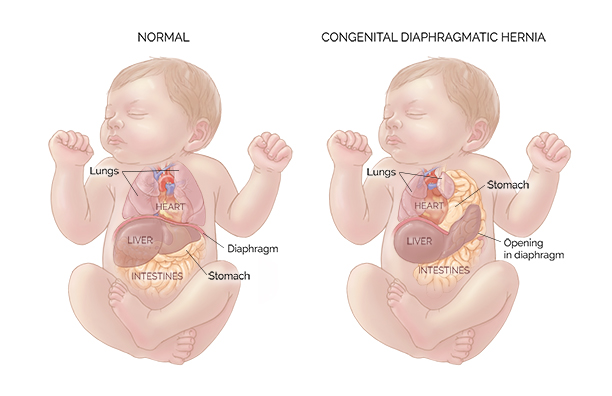
All CDH are treated surgically; however, the severity of the disease determines what surgical approach will be used. In mild or moderate cases, primary repair and muscle flap repair are used. Primary repair consists of suturing the hernia close, and muscle flap repair consists of taking a section of the abdominal muscle and using it to cover the hernia. Both of these techniques are appropriate only when the native diaphragmatic muscle is present. In most severe cases, when the child has no diaphragmatic muscle present, a surgeon will use the tension-free suturing technique in which a soft-tissue mesh is sutured into the patient [4].
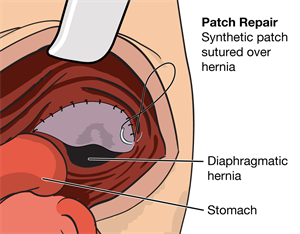
“A better patch is the next incremental step in improving survival rates in CDH.”
Dr. Patricia Chess (Neonatologist)
Current Problem
51% of CDH cases are repaired with a mesh, and re-herniation occurs in 41-46% of the cases [6]. Moreover, the literature suggests that re-herniation is 2.83 times higher in tension-free surgical repair in comparison to primary and muscle flap repair [7]. A typical complication of hernia mesh usage is product failure related to the material properties of the mesh which lead to the INABILITY OF SYNTHETIC MESHES TO EXPAND.
Extruded woven or knitted pattern of the synthetic polymers CONTRACT and HARDEN, leading to mesh shrinkage, forcing the mesh to PULL OUT of the tissue and MIGRATE [8].
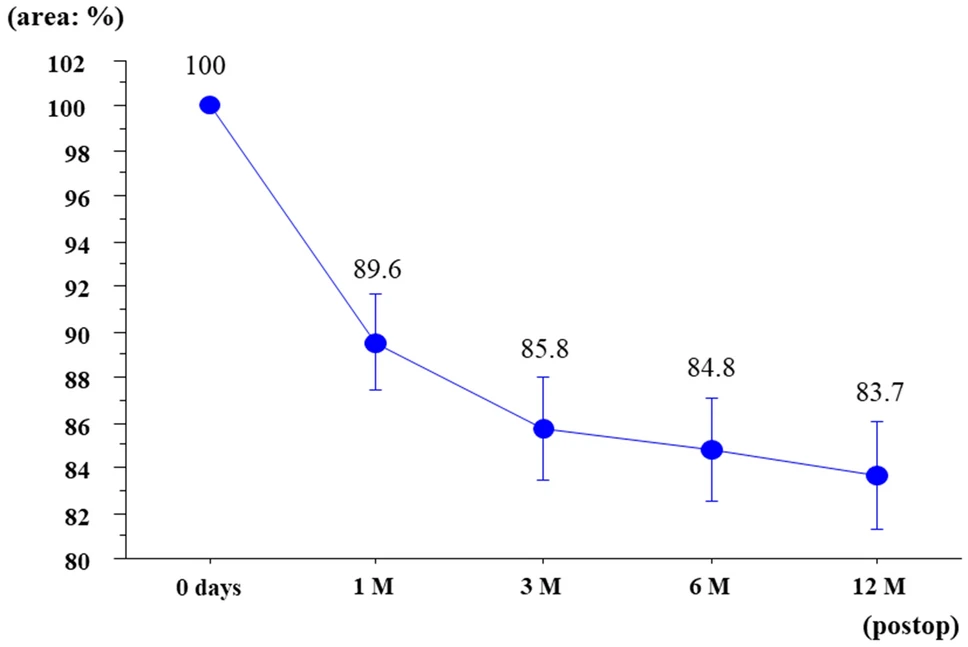
Currently, the most common patch used to treat CDH is the Gore-Tex Soft Tissue patch, as seen in Figure 3. Gore-Tex Soft Tissue patch is made of ePTFE which has a high tensile strength, low porosity, is biocompatible, and can be trimmed to size without losing its material properties. However, the Gore-Tex patch is not perfect. The main issue is that the patch cannot expand once implanted which causes it pull away from the muscle and causes re-herniation. Some of the other limitations include that it is currently being used off-label as it is not cleared by the FDA to treat CDH. Studies also suggest that the porosity of the patch might lead to excessive inflammatory response which then causes the material to contract up to 50% upon implantation which leads to further occurrences of re-hernation.
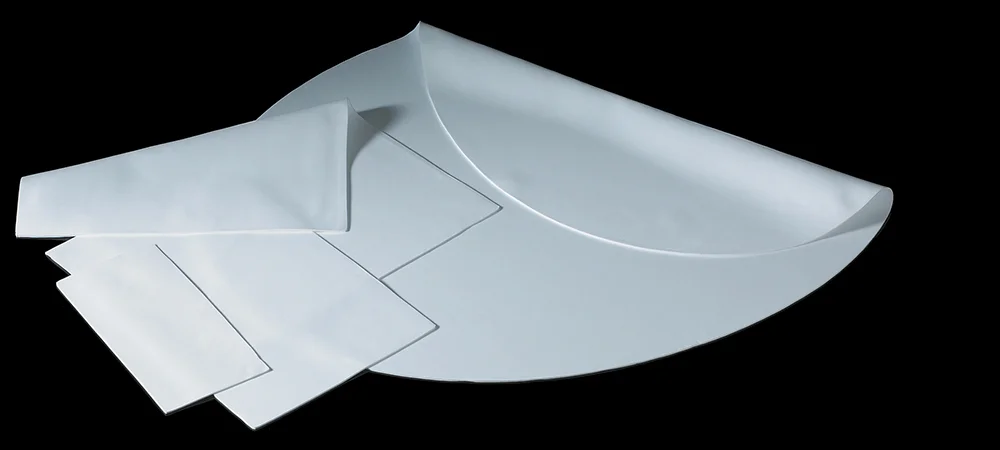
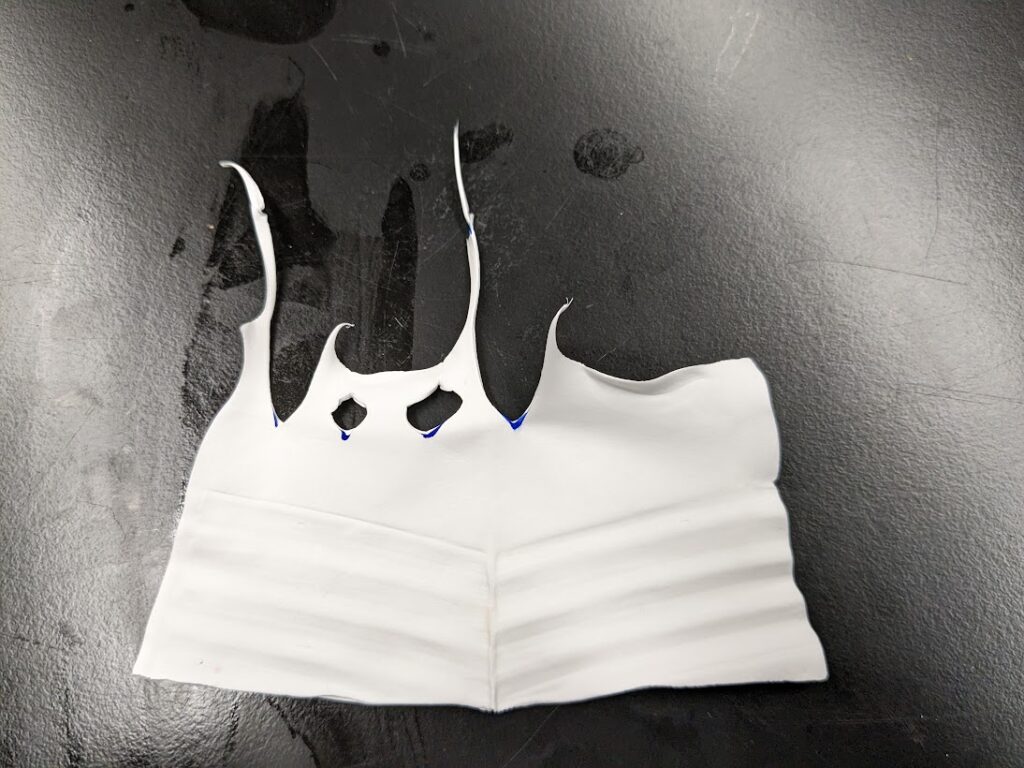
Figure 4. Above is pictured the Gore-Tex Soft Tissue Patch. On the left is shown a Gore-Tex Soft Tissue Patch in different sizes. On the right is shown the patch tested to failure at 90 N.
The three ways a patch could fail and cause re-herniation are if the mesh itself ripped, the sutures securing the patch to the diaphragm rip, or if the sutures pull out of the diaphragm and rip the diaphragm. Through testing we found that the current technology, the Gore-Tex 1mm patch, ripped at 90N and sutures, 2-0 Ethibond, rip at 60N. Then through a literature search we found that the pull out force of sutures in skeletal muscle is approximately 40N [9]. Therefore, currently re-herniation is called by the sutures pulling out of the diaphragm, so in order to avoid re-herniation our mesh needs to expand with a force less than 40N.
Component | Force to Break |
Mesh (Gore-Tex 1mm Patch) | 90 N |
Suture (2-0 Ethibond) | 60 N |
Diaphragm (Suture Pull Out Force) | 40 N |
“You’re repairing a muscle which means it must be a strong repair, and your repair must grow and be able to persist with the child as the kid grows.”
Dr. Nicole Wilson (Pediatric Surgeon)
Our Product
Our team created a hernia mesh that expands gradually, allowing the device to grow in response to the changing size requirements of the child. The gradual expansion is meant to reduce the tension applied to the mesh, decreasing the probability for the mesh to migrate and for re-herniation to occur. Our patent-pending technology includes a cross linked back bone of sine waves made of durable and flexible TPU and a soft casing made of silicone. The sine pattern of the backbone was chosen as it allowed quick and easy changing of parameters (amplitude and frequency) and therefore quick and easy prototyping. Further changes were made by “clipping” the tips of the sine waves to create a more gentle curve. At certain points and in a grid like pattern, nodes were placed, allowing for proper and complete unison of the two layers.
The two layers are of the same design, with one layer oriented 90 degrees to the other. This allows for proper expansion in 2 directions in unison with each other. The ends of the mesh have “arched” structures, connecting the sine wave elements together and giving surgeons specified suture points to allow for proper placement on the diaphragm. This final backbone was then placed in a mold where liquid silicone of Shore00-10 hardness (very, very soft silicone) was added. This gave an airtight casing to the backbone, creating a patch that is strong, airtight, and expandable.
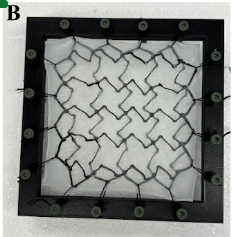
Key Requirements and Specifications
Our key requirements and specifications are listed in Table 2 below. They are based on testing, literature searches, and conversations with clinicians. The most important metric is that our mesh can expand before 40N of force is applied to it. The pull out force of sutures in the diaphragm is 40N so our patch must fully expand before that point in order to prevent reherniation.
Requirement | Specification |
Size of Patch | 6.5 cm in diameter |
Shelf Life | ASTM F1980 |
Biocompatibility | ISO 10993 |
Growth | 2.5x of Original Size |
Withstand Pressure | 11 kPa |
Sterilization | ISO 11607 and ISO 11737 |
Coefficient of Friction | 0.13 |
Suture Margin | 1.5 cm |
Withstand Force | Expand before 40 N |
Testing and Justifications
Stretch and Force Test
The goal of our patch was to expand 2.5x in order to meet the growing size of the diaphragm in children. It also needs to expand to its maximum length under 40N, as that is the force that the sutures pull out of the diaphragm currently and causes reherniation. We therefore stretched our mesh from 4x4cm to 10x10cm and measured the force required. We also compared this to a silicone sheet without a backbone for comparison to showcase the need for the backbone.
Our patch stretched 2.5x with an average of 27.1 +/- 2.4 N of force. The patch without a backbone failed before stretching all the way, failing at 10.3 N of force. Therefore, the inclusion of a backbone embedded in the mesh is necessary to withstand the stretching required in our mesh.
Deformation Test
The purpose of this test was to demonstrate that NeoMesh withstands the pressure of the pleural cavity in conditions such as respiration and coughing without experiencing failure and extreme deformation after achieving 2.5x elongation (Figure 6). Moreover, this test was used to prove that our cutting-edge sinusoidal backbone is needed to achieve optimal conditions for the mesh. Literature shows that the maximum trans-diaphragmatic pressure is 11kPa [11], thus our mesh must be able to withstand this pressure.
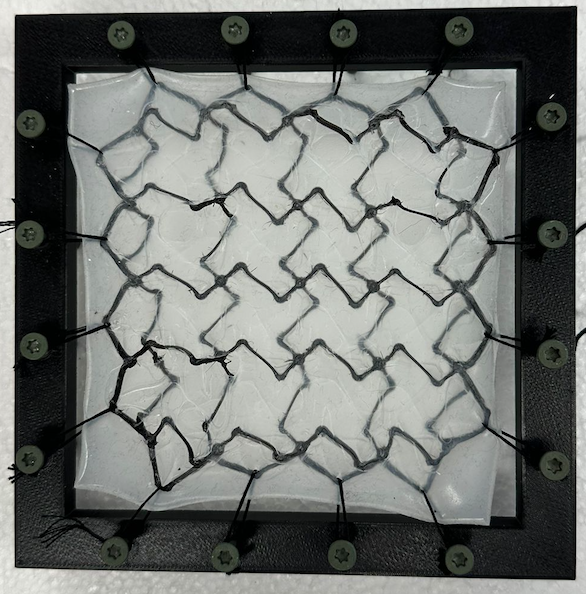
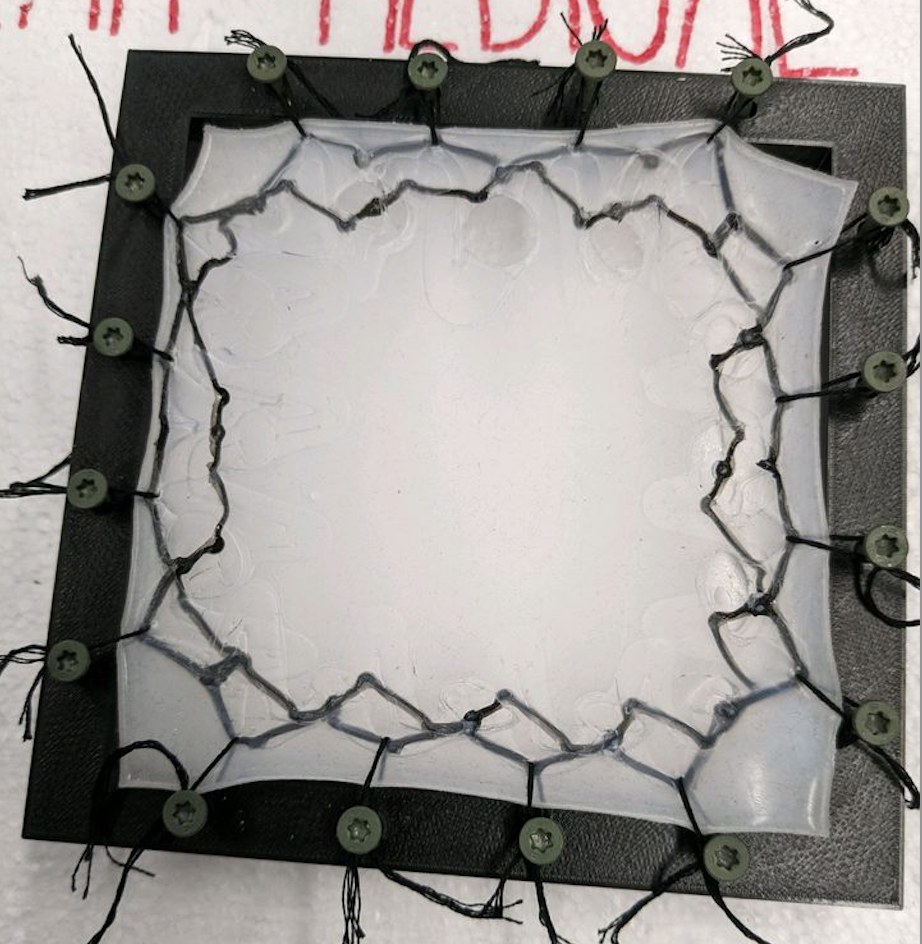
Figure 6. A – Mesh with backbone expanded to 2.5x B – Mesh without backbone expanded to 2.5x
The meshes were subjected to pressures greater than 11 kPa, and the deformation of the meshes were measured in cm. A statistical analysis was used and it was determined that there was a significant difference between the deformation for each mesh. Moreover, as seen in Figure 7, the deformation for the mesh without a backbone was much higher than that of the NeoMesh. This proves that the backbone is necessary to prevent the mesh from collapsing into the thoracic or abdominal cavity.
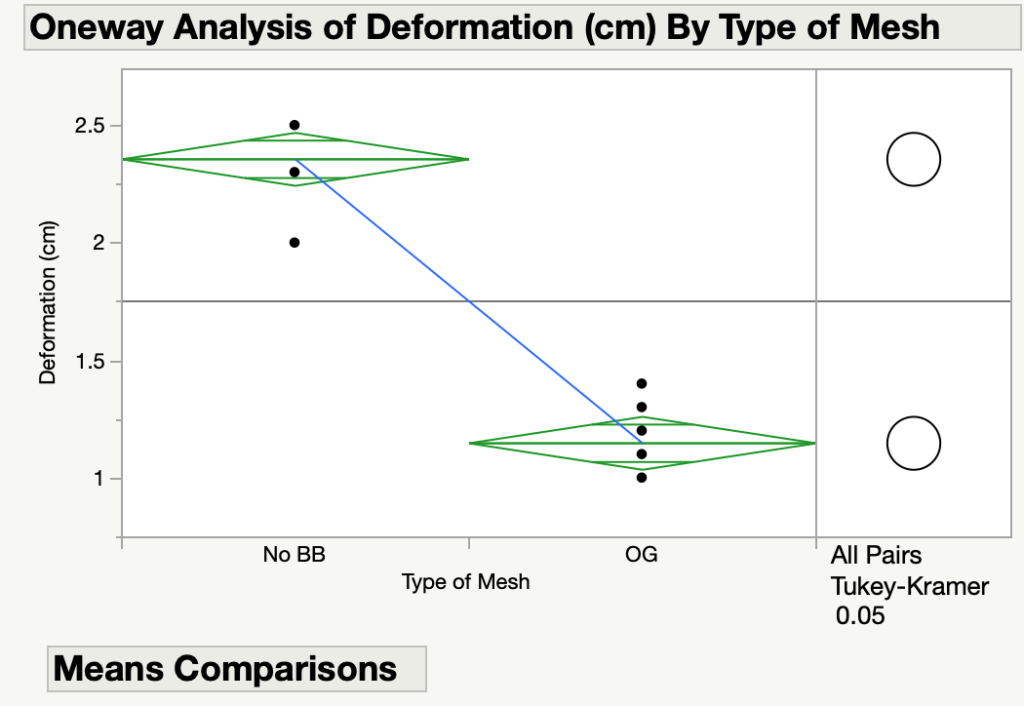
Limitations and Future Considerations
Limitation – Market Size
While our focus was to create an expandable mesh to reduce the occurrence of reherniation in children with CDH, it must be noted the difficulty of taking our product to market. There are 1,400 cases of CDH per year in the US, and of those there are 50 severe cases in which a patch would be used. We believe it was still a worthy endeavor to tackle this issue, but the small number of cases per year would not be able to support a business. Therefore our most likely path forward would be to patent our design and then license the technology to current hernia mesh manufacturers such as Gore Medical.
Future Consideration – Further Testing
Our product would require further testing before going to market. First we would to verify that it meets our requirements and specifications. This includes adhering to FDA and ISO standards such as ISO 10993 for biocompatibility, ASTM F1980 for shelf life, and ISO 11607 and ISO 11737 for sterilization. We would also need to preform heuristics testing as our mesh
Future Consideration – Materials
Currently our prototype mesh is made of two components: the 3D printed sinusoidal backbone and the silicone enclosure around it. The 3D printed part is made of TPU filament and the enclosure is Smooth-On EcoFlex 00-10 which is a platinum cure silicone with a durometer rating of 00-10. These are the materials for our prototype though, and our final product would need to have biocompatible materials. We have identified medical grade TPU and silicone that could be used in the final product, though further testing would also need to be done to ensure the material properties remain the same.
Future Consideration – Manufacturing
Currently our mesh is manufactured by 3D printing two identical meshes, gluing the individual nodes together to create a two layer mesh, and then encasing them in a bed of silicone. This is a very time intensive and hands-on process to create a single mesh. Therefore, we would outsource our manufacturing of the final product to OEM to increase productivity and decrease time to manufacture.
Acknowledgements
We would like to thank the people who have made this project possible!
Dr. Greg Gdowski – CMTI Executive Director
Dr. Amy Lerner – CMTI Academic Director
Dr. Nicole Wilson – Pediatric Surgeon
Marty Gira – CMTI Senior Research Engineer
Mahllet Beyene – CMTI Graduate Program Coordinator
Dr. Patricia Chess – Neonatologist